Physicists Pinpoint Elementary Particle, Leading Way to Higgs
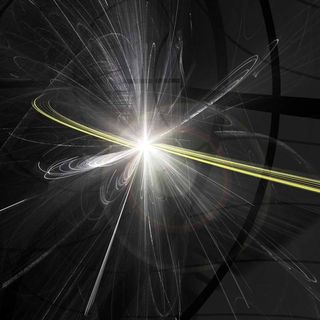
Physicists at Fermilab in Chicago have improved the measurement of a subatomic particle called the W boson. Their result won't just help physicists better understand exotic particles; it also narrows the range of possible energies of W’s flashier cousin the Higgs boson, dubbed the "God particle" in the media.
To obtain their new-and-improved value for the W boson mass, physicists working on the CDF (Collider Detector at Fermilab) experiment analyzed data from hundreds of trillions of particle collisions inside the Tevatron, a particle accelerator at Fermilab. The Tevatron no longer plays pingpong with particles — it shut down for good last fall — but this trove of data was gathered in the four years before its retirement.
The CDF team announced the new results at a seminar this morning (Feb. 23). As it turns out, the W boson has an energy (also equivalent to mass) of 80.387 billion electron-volts, or giga-electron volts (GeV), plus or minus 19 million electron-volts — a narrower margin of error than existed previously. (For comparison, a proton has a mass of about 0.938 GeV). The new level of certainty reduces the upper bounds of the Higgs' mass from 161 GeV to 145 GeV. [Gallery: Search for the Higgs Boson]
Spokesman Rob Roser explained how his team translated particle collisions into hard numbers.
"In our world we collide protons and antiprotons together, which means we're colliding three quarks inside the protons with three anti-quarks [inside the antiprotons], and sometimes this makes a W boson," Roser told LiveScience. Quarks are the building blocks of protons, and anti-quarks are their antimatter equivalents.
However, when the three quarks collide with the three anti-quarks, only two of the particles — the ones in front, so to speak — actually bash together, and so scientists don't know the full story of how energy was distributed in the collision. To get around this problem, Roser said he and his colleagues must average out the results of trillions of proton-antiproton bombardments, and choose the best W boson production events from among the lot. They then compare these events to computer-simulated templates of what the W mass could be. "We see which one matches best."
Roser said that knowing the mass of the W boson is an important number in the Standard Model — the body of laws that describes the dynamics between subatomic particles. "It gets used everywhere in terms of calculations," Roser said. When physicists try to understand the nature of supersymmetry, prove the existence of a particle called the Z boson, or calculate particle interactions, they call upon the mass of W.
Sign up for the Live Science daily newsletter now
Get the world’s most fascinating discoveries delivered straight to your inbox.
Supersymmetry suggests that in addition to all known particles, supersymmetric partner particles exist. Called “sparticles,” these differ from their "normal" partners by half a unit of spin, one of the fundamental characteristics of elementary particles. [The Coolest Little Particles in Nature]
"This is an important piece of the puzzle and we know it better than we've ever known it," he said.
The mass of the W boson is also useful by way of its special relationship with the elusive Higgs particle. The masses of the W boson, Higgs boson and the so-called top quark, one of the six flavors of quarks, have a "linear relationship," Roser explained; knowing the exact value of any two of them means you can calculate the value of the third. The mass of the top quark is known within a margin of error of 1 percent, and the new improvement in the measurement of the mass of the W boson has therefore tightened the range in possible values of the mass of the Higgs.
Also known as the "God particle," the Higgs boson is thought to generate a field that permeates space and imbues all the matter in the universe with mass. Physicists are hot on its trail at CERN Laboratory in Switzerland, but it hasn't yet been positively identified. The CDF team will announce the results of its own direct search for the Higgs particle in two weeks.
Follow Natalie Wolchover on Twitter @nattyover. Follow LiveScience for the latest in science news and discoveries on Twitter @livescience and on Facebook.
Natalie Wolchover was a staff writer for Live Science from 2010 to 2012 and is currently a senior physics writer and editor for Quanta Magazine. She holds a bachelor's degree in physics from Tufts University and has studied physics at the University of California, Berkeley. Along with the staff of Quanta, Wolchover won the 2022 Pulitzer Prize for explanatory writing for her work on the building of the James Webb Space Telescope. Her work has also appeared in the The Best American Science and Nature Writing and The Best Writing on Mathematics, Nature, The New Yorker and Popular Science. She was the 2016 winner of the Evert Clark/Seth Payne Award, an annual prize for young science journalists, as well as the winner of the 2017 Science Communication Award for the American Institute of Physics.