8 ways you can see Einstein's theory of relativity in real life
Relativity is one of the most famous scientific theories of the 20th century, but how well does it explain the things we see in our daily lives?
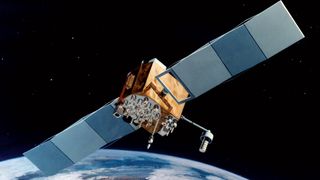
Albert Einstein began formulating the theory of relativity in 1905 to explain the behavior of objects in space and time, and the groundbreaking work can be used to predict things such as the existence of black holes, light bending due to gravity and the behavior of planets in their orbits.
The theory is deceptively simple. First, there is no "absolute" frame of reference: Every time you measure an object's velocity, its momentum or how it experiences time, it's always in relation to something else. Second, the speed of light is the same no matter who measures it or how fast the person measuring it is going. Third, nothing can go faster than light.
Einstein's most famous theory has profound implications. If the speed of light is always the same, it means that an astronaut going very fast relative to Earth will measure the seconds ticking by more slowly than an Earthbound observer will. Time essentially slows down for the astronaut — a phenomenon called time dilation.
Related: What would happen if the speed of light was much lower?
Any object in a big gravity field accelerates, so it also experiences time dilation. Meanwhile, the astronaut's spaceship experiences length contraction, which means if you took a picture of the spacecraft as it flew by, it would look as though it were "squished" in the direction of motion. To the astronaut on board, however, all would seem normal. In addition, the mass of the spaceship would appear to increase from the point of view of people on Earth.
But you don't necessarily need a spaceship zooming at near light speed to see relativistic effects. There are several instances of relativity we can see in our daily lives and technologies we use today that demonstrate Einstein was right. Here are some common examples of the theory of relativity in action.
Electromagnets
Magnetism is a relativistic effect, and you can see this demonstrated in generators. If you take a loop of wire and move it through a magnetic field, you generate an electric current. The charged particles in the wire are affected by the changing magnetic field, which forces some of them to move and creates the current.
But now, picture the wire at rest and imagine the magnet is moving. In this case, the charged particles in the wire (the electrons and protons) aren't moving anymore, so the magnetic field shouldn't be affecting them. But it does, and a current still flows. This shows that there is no privileged frame of reference.
Thomas Moore, a professor of physics at Pomona College in Claremont, California, uses the principle of relativity to demonstrate Faraday's law, which states that a changing magnetic field creates an electric current.
"Since this is the core principle behind transformers and electric generators, anyone who uses electricity is experiencing the effects of relativity," Moore told Live Science.
Thomas Moore is a theoretical astrophysicist at Pomona College in Claremont, California. His research has mainly focused on the generation and detection of gravitational waves. He has published a number of books and articles, including "Six Ideas That Shaped Physics" (3rd Edition, McGraw-Hill, 2017) and "A General Relativity Workbook" (University Science Books, 2013).
Electromagnets work via relativity as well. When a direct current of electric charge flows through a wire, electrons drift through the material. Ordinarily, the wire would seem electrically neutral, with no net positive or negative charge, because the wire has about the same number of protons (positive charges) and electrons (negative charges). But if you put another wire with a direct current next to it, the wires attract or repel each other, depending on the direction in which the current is moving, according to physicists at the University of Illinois at Urbana-Champaign.
Assuming the currents are moving in the same direction, the electrons in the second wire are motionless compared to the electrons in the first wire. (This assumes the currents are about the same strength.) Meanwhile, the protons in both wires are moving in comparison to the electrons in both wires. Because of the relativistic length contraction, they appear to be more closely spaced, so there's more positive charge than negative charge per length of wire. Because like charges repel, the two wires also repel.
Currents in the opposite directions result in attraction, because compared to the first wire, the electrons in the other wire are more crowded, thus creating a net negative charge, according to the University of Illinois at Urbana-Champaign. Meanwhile, the protons in the first wire are creating a net positive charge, and opposite charges attract.
GPS navigation
For your car's GPS navigation to function as accurately as it does, satellites have to consider relativistic effects, according to PhysicsCentral. This is because even though satellites aren't moving anywhere close to the speed of light, they are still going pretty fast. The satellites are also sending signals to ground stations on Earth. These stations (and the GPS technology in a car or smartphone) are all experiencing higher accelerations due to gravity than the satellites in orbit.
To get that pinpoint accuracy, the satellites use clocks that are accurate to a few nanoseconds (billionths of a second). Because each satellite is 12,600 miles (20,300 kilometers) above Earth and moves at about 6,000 mph (10,000 km/h), there's a relativistic time dilation that tacks on about 4 microseconds each day. Add in the effects of gravity, and the time dilation effect goes up to about 7 microseconds (millionths of a second).
The difference is very real: If no relativistic effects were accounted for, a GPS unit that tells you it's a half mile (0.8 km) to the next gas station would be 5 miles (8 km) off after only one day, according to Physics Central.
Gold's yellow color
Most metals are shiny because the electrons in the atoms jump from different energy levels, or "orbitals." Some photons that hit the metal get absorbed and reemitted, though at a longer wavelength. However, most visible light gets reflected.
Gold is a heavy element, so the inner electrons move fast enough that the relativistic mass increase and the length contraction are significant, according to a statement from Heidelberg University in Germany. As a result, the electrons spin around the nucleus in shorter paths, with more momentum. Electrons in the inner orbitals carry energy that is closer to the energy of outer electrons, and the wavelengths that get absorbed and reflected are longer. Longer wavelengths of light mean that some of the visible light that would usually be reflected gets absorbed, and that light is on the blue end of the spectrum. White light is a mix of all the colors of the rainbow, but in gold's case, when light gets absorbed and reemitted, the wavelengths are usually longer. That means the mix of light waves we see tends to have less blue and violet in it. Because yellow, orange and red light are longer wavelengths than blue light, gold appears yellowish, according to the BBC.
Gold's resistance to corrosion
The relativistic effect on gold's electrons is also one reason it doesn't corrode or easily react with anything else, according to a 1998 paper in the journal Gold Bulletin.
Gold has only one electron in its outer shell, but it still is not as reactive as calcium or lithium. Instead, because the electrons in gold are "heavier" than they should be, since they are moving near the speed of light, increasing their mass, they are held closer to the atomic nucleus. This means that the outermost electron isn't likely to be where it can react with anything at all; it's just as likely to be among the electrons that are close to the nucleus.
Liquid mercury
Mercury is also a heavy atom, with electrons held close to the nucleus because of their speed and consequent mass increase. The bonds between mercury atoms are weak, so mercury melts at lower temperatures and is typically a liquid when we see it, according to Chemistry World.
Your old TV
Until about the early 2000s, most televisions and monitors had cathode ray tube screens. A cathode ray tube works by firing electrons at a phosphor surface with a big magnet. Each electron makes a lighted pixel when it hits the back of the screen, and the electrons fire out to make the picture move at up to 30% the speed of light. Relativistic effects are noticeable, and when manufacturers shaped the magnets, they had to consider those effects, according to PBS News Hour.
Light
Isaac Newton assumed that there is an absolute rest frame, or an external perfect frame of reference that we could compare all other frames of reference against. If he had been right, we would have to come up with a different explanation for light, because it wouldn't happen at all.
"Not only would magnetism not exist, but light would also not exist, because relativity requires that changes in an electromagnetic field move at a finite speed instead of instantaneously," Moore said. "If relativity did not enforce this requirement … changes in electric fields would be communicated instantaneously … instead of through electromagnetic waves, and both magnetism and light would be unnecessary."
The sun
Without Einstein's most famous equation — E = mc^2 — the sun and the rest of the stars wouldn't shine. In the center of our parent star, intense temperatures and pressures constantly squeeze four separate hydrogen atoms into a single helium atom, according to Ohio State University. The mass of a single helium atom is just slightly less than that of four hydrogen atoms. What happens to the extra mass? It gets directly converted into energy, which shows up as sunlight on our planet.
Additional resources
- Learn more about how relativity affects the study of distant cosmic objects, from NASA.
- See how relativity becomes important for particle accelerators, from Fermilab.
- Explore a history of Einstein's theory of relativity and its many confirmed predictions, from the European Space Agency.
Sign up for the Live Science daily newsletter now
Get the world’s most fascinating discoveries delivered straight to your inbox.
- Adam MannLive Science Contributor