Greatest Mysteries: What Happens Inside an Earthquake?
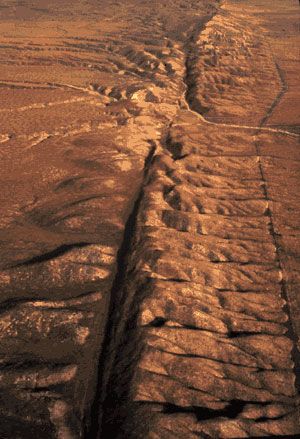
Editor's Note: We asked several scientists from various fields what they thought were the greatest mysteries today, and then we added a few that were on our minds, too. This article is one of 15 in LiveScience's "Greatest Mysteries" series running each weekday.
When a sizable earthquake strikes, experts can explain exactly where it started and what type of fault is involved and maybe even predict how long aftershocks will last. But the strange truth is that seismologists and geophysicists are quite unsure of what happens inside the planet during a quake. Earthquake physics has undergone a revolution during the past decade, thanks to new insights from lab experiments, field studies of exhumed faults and better theories. But the nature and behavior of the forces that keep faults from moving and then suddenly fail are still unknown. And when faults do move, something is missing—there is little to no evidence of the extremely high levels of friction and melting that would be expected to follow above ground when two giant rocks slid against each other. "There are many reasons to believe that something exotic is happening," said Caltech geophysicist Tom Heaton. "The problem of frictional sliding in earthquakes is one of the most fundamental problems in all of Earth science," Heaton said. "It has been a 30-year mystery story of figuring out the basic physics of the earthquake problem." Gentle earthquakes Most earthquakes happen where tectonic plates meet and glide against each other. Quakes occur when the frictional stress of the movement exceeds the strength of the rocks, causing a failure at a fault line. Violent displacement of the Earth's crust follows, leading to a release of elastic strain energy. This energy takes the form of shock waves that radiate and constitute an earthquake. One of the strangest things about earthquakes is how gentle they are, Heaton said. For instance, some scientists thought they had figured out how to simulate mini-earthquakes in the lab. But when they scale up the energies observed in the lab to the size of real faults, the model would predict extensive melting on faults. And such models predict devastation far beyond what killed more than 500 people this week in Peru, more than 80,000 people in the 2005 Pakistan quake or more than a quarter of a million people in the 2004 earthquake off the coast of Sumatra. "Earthquakes would be so violent that no living thing could survive the shaking," Heaton said. Therefore, no one has actually simulated anything close to a real earthquake yet. A machine design problem The simulation problem lies partly in the fact that it's very difficult to make lab machines generate all the environmental conditions that occur miles below ground during an earthquake—including high stress, high pressure, elevated temperatures and a slip rate of about a yard per second (about the pace at which we walk). David Goldsby and his rock mechanics colleagues at Brown University have designed machines that can apply the high stresses of temblors to rock specimens so the geophysicists can study friction at depth. "We can apply normal stresses as high as occur throughout the entire seismogenic zone of the Earth's crust, about 10 kilometers [6 miles] in depth," he said. That's incredibly impressive and important for earthquake science, but it still leaves a lot of questions unanswered, because what happens inside the Earth is so strange in magnitude and physics. "No apparatus in the world is yet capable of meeting all of these criteria," Goldsby said. Normal friction Above ground, friction is a steady, stubborn force that opposes motion. Friction generates heat, as people with cold hands know, and increases with the stress you put on objects. So the heat on faults during sliding should increase with depth in the Earth. The rocks should definitely melt where they meet. But underground, during earthquakes, two huge, hard, weight-pressurized rock slabs slip past or under each other. And nothing melts. Usually. That's weird. It could be because the friction and thus the heat are much lower than you'd expect from rocks above ground, Goldsby said. Earthquake friction works like this, Heaton said: It starts out high when there is little to no movement; then friction plummets to zero as the rocks move fast; then friction goes to high again when the rocks slow down. That weird behavior of friction during an earthquake might be the reason there is little to no melting, Goldsby said. If friction is low when the rocks move fast, then much less heat is generated and no detectable melting occurs. Maybe some other mechanism kicks in before the rocks get to their melting phase, Heaton said. One explanation is "flash heating." Faults are stuck in place by very high forces. Once faults start sliding, if they slide fast enough, they become extra slippery at microscopic contact points, like skaters on ice. Heat is generated, but the result is a zero-friction, high-temperature cushiony flash of light or superheated gas called plasma that yields no detectable melted material, Heaton said. When the faults slow down, they stick tight again. Another idea is that pressurized water in the rocks during a slip could decrease the stress on the fault and therefore the friction, Goldsby said. Faults might ride on a cushion of steam, allowing the fault to slide at low friction and the rock heat would not reach the melting point. Ripple in the rug The key to understanding earthquakes is actually not where they start but how the fracture spreads, and that has a lot to do with the weird behavior of underground friction, Heaton says. The highest sliding velocities happen at the leading edge of a pulse of slip that runs through the Earth like a ripple in a rug, says Heaton, who described this fault behavior in a landmark paper 17 years ago. Think of a fault as a rug that you want to move, he said. You can just pull the rug from the edge. That's the hard way to move it. The easy way to move a rug is to "put a little bump in it and move the bump and when you're done, you've offset the rug," he explained. Friction is in a yin-yang arrangement with those slip-pulses, it turns out, Heaton said. "The slip in the pulse depends on the friction, but it turns out the friction turns on how fast the slip is happening," he said. "That's a math problem, a positive feedback system. They are notoriously unstable." If you knew how big a pulse would be, you could predict an earthquake's magnitude, but the exotic behavior of friction underground botches all that up, Heaton said. Still, the revolution in the field of earthquake physics has brought new insights, Goldsby said. "I am not only hopeful but certain that we will learn even more about how earthquakes occur in the coming decade," he said. "This knowledge will help us understand how to mitigate the damaging effects of earthquakes and help prevent the loss of life, and may someday allow us to detect earthquake precursors."
- Another Great Mystery: What Drives Evolution?
- Image Gallery: Deadly Earthquakes
- Life's Little Mysteries
Sign up for the Live Science daily newsletter now
Get the world’s most fascinating discoveries delivered straight to your inbox.
Robin Lloyd was a senior editor at Space.com and Live Science from 2007 to 2009. She holds a B.A. degree in sociology from Smith College and a Ph.D. and M.A. degree in sociology from the University of California at Santa Barbara. She is currently a freelance science writer based in New York City and a contributing editor at Scientific American, as well as an adjunct professor at New York University's Science, Health and Environmental Reporting Program.