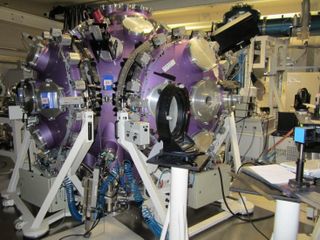
This story was updated on Oct. 9 at 2:20 p.m. ET
The daydream of science-fiction fans and supervillains everywhere has inched one step closer to reality: Scientists have demonstrated a new technique for nuclear fusion, the process that fuels stars like the sun, that doesn't produce hazardous particles.
The new experiment coaxed a boron atom to fuse with a hydrogen nucleus, using a little help from incredibly powerful laser and proton beams. The fusion produced alpha particles, which are more easily converted to usable energy than the high-energy neutrons produced by prior fusion methods.
High-energy neutrons can also produce radiation if they fuse with other nuclei to form radioactive elements.
Elusive dream
In nuclear fusion, heat and pressure force two atoms to overcome their intense repulsion to form one atom, releasing a huge amount of energy in the process. For 50 years, scientists have chased the dream of producing limitless, clean energy from nuclear fusion. [Science Fact or Fiction? The Plausibility of 10 Sci-Fi Concepts]
"This is really the Holy Grail," said study co-author Christine Labaune, a physicist for the École Polytechnique in France.
Sign up for the Live Science daily newsletter now
Get the world’s most fascinating discoveries delivered straight to your inbox.
Fusion is the engine that powers the sun and other stars: At the sun's incredibly dense core, the pressure of the surrounding gas fuses hydrogen atoms together, forming helium.
But here on Earth, fusion requires incredibly hot temperatures, sometimes in excess of 100 million degrees Celsius (180 million degrees Fahrenheit). Most efforts have focused on fusing deuterium and tritium, heavy forms of hydrogen, to form a helium atom, because that reaction can occur at high rates even at relatively cool temperatures. But the process also releases high-energy neutrons, which must be contained by heavy-duty shielding material, which then becomes radioactive when it interacts with the neutrons.
What's more, after 40 years of efforts, the deuterium-tritium reaction hasn't quite reached the break-even point, in which the amount of energy produced by the fusion reaction is equal to the energy put into it, said Peter Thirolf, a physicist at the Ludwig-Maximilian-University of Munich in Germany who was not involved in the study. (Scientists at the National Ignition Facility in Livermore, Calif., recently announced that they are very close to nuclear fusion's break-even point, and that the barriers to achieving it are engineering-related, rather than physics-related.)
New technique
But Labaune and her colleagues have chosen to focus instead on completely different fusion reactions. Taking advantage of the fact that lasers have gotten ever more powerful over the years, the team briefly pulsed a focused laser beam with incredibly high energy at a plasma of boron-11, an isotope of boron with an extra neutron. Meanwhile, another intense proton beam bombarded the boron plasma from another direction.
The boron isotopes fused with the laser-driven protons to produce beryllium and alpha particles, which are made up of two protons and two neutrons bound together — a key signature of the fusion reaction. The new experiment has already produced orders of magnitude more energy than a past experiment with boron fusion. And unlike high-energy neutrons, the alpha particle energy can be contained easily and converted into electrical current that could then be used in other processes, Labaune said.
The experiment is an exciting step, but it's still a proof of principle, Thirolf said. Even on a small scale, however, it could eventually prove useful to study the fusion processes churning at the hearts of stars, he added.
Given the new method's early stage of development, there are also many opportunities for improvements, Thirolf said.
But large-scale nuclear fusion is still a distant reality.
"When I started as a student, people said, 'We will get the fusion reactor in 30 years,'" Thirolf told LiveScience. "What I'm telling my students now is, 'We will get the fusion reactor in 30 years.'"
The technique was described today (Oct. 8) in the journal Nature Communications.
Editor's Note: This story was updated to correct the conversion of Celsius to Fahrenheit. 100 million degrees Celsius is 180 million degrees Fahrenheit.
Follow Tia Ghose on Twitter and Google+. Follow LiveScience @livescience, Facebook & Google+. Original article on LiveScience.
Tia is the managing editor and was previously a senior writer for Live Science. Her work has appeared in Scientific American, Wired.com and other outlets. She holds a master's degree in bioengineering from the University of Washington, a graduate certificate in science writing from UC Santa Cruz and a bachelor's degree in mechanical engineering from the University of Texas at Austin. Tia was part of a team at the Milwaukee Journal Sentinel that published the Empty Cradles series on preterm births, which won multiple awards, including the 2012 Casey Medal for Meritorious Journalism.