
An End in Sight in the Long Search for Gravity Waves (Op-Ed)
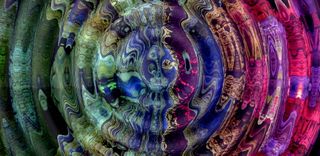
This article was originally published at The Conversation. The publication contributed the article to Live Science's Expert Voices: Op-Ed & Insights.
Our unfolding understanding of the universe is marked by epic searches and we are now on the brink of discovering something that has escaped detection for many years.
The search for gravity waves has been a century long epic. They are a prediction of Einstein’s General Theory of Relativity but for years physicists argued about their theoretical existence.
By 1957 physicists had proved that they must carry energy and cause vibrations. But it was also apparent that waves carrying a million times more energy than sunlight would make vibrations smaller than an atomic nucleus.
Building detectors seemed a daunting task but in the 1960s a maverick physicist Joseph Weber, at the University of Maryland, began to design the first detectors. By 1969 he claimed success!
There was excitement and consternation. How could such vast amounts of energy be reconciled with our understanding of stars and galaxies? A scientific gold rush began.
Within two years, ten new detectors had been built in major labs across the planet. But nothing was detected.
Sign up for the Live Science daily newsletter now
Get the world’s most fascinating discoveries delivered straight to your inbox.
Going to need a better detector
Some physicists gave up on the field but for the next 40 years a growing group of physicists set about trying to build vastly better detectors.
By the 1980s a worldwide collaboration to build five detectors, called cryogenic resonant bars, was underway, with one detector called NIOBE located at the University of Western Australia.
These were huge metal bars cooled to near absolute zero. They used superconducting sensors that could detect a million times smaller vibration energy than those of Weber.
They operated throughout much of the 1990s. If a pair of black holes had collided in our galaxy, or a new black hole had formed, it would have been heard as a gentle ping in the cold bars… but all remained quiet.
What the cryogenic detectors did achieve was an understanding of how quantum physics affects measurement, even of tonne-scale objects. The detectors forced us to come to grips with a new approach to measurement. Today this has grown into a major research field called macroscopic quantum mechanics.
But the null results did not mean the end. It meant that we had to look further into the universe. A black hole collision may be rare in one galaxy but it could be a frequent occurrence if you could listen in to a million galaxies.
Laser beams will help
A new technology was needed to stretch the sensitivity enormously, and by the year 2000 this was available: a method called laser interferometry.
The idea was to use laser beams to measure tiny vibrations in the distance between widely spaced mirrors. The bigger the distance the bigger the vibration! And an L-shape could double the signal and cancel out the noise from the laser.
Several teams of physicists including a team at the Australian National University had spent many years researching the technology. Laser beam measurements allowed very large spacing and so new detectors up to 4km in size were designed and constructed in the US, Europe and Japan.
The Australian Consortium for Gravitational Astronomy built a research centre on a huge site at Gingin, just north of Perth, in Western Australia, that was reserved for the future southern hemisphere gravitational wave detector.
The world would need this so that triangulation could be used to locate signals.
Latest detectors
The new detectors were proposed in two stages. Because they involved formidable technological challenges, the first detectors would have the modest aim of proving that the laser technology could be implemented on a 4km scale, but using relatively low intensity laser light that would mean only a few per cent chance of detecting any signals.
The detectors were housed inside the world’s largest vacuum system, the mirrors had to be 100 times more perfect than a telescope mirror, seismic vibrations had to be largely eliminated, and the laser light had to be the purest light ever created.
A second stage would be a complete rebuild with bigger mirrors, much more laser power and even better vibration control. The second stage would have a sensitivity where coalescing pairs of neutron stars merging to form black holes, would be detectable about 20 to 40 times per year.
Australia has been closely involved with both stages of the US project. CSIRO was commissioned to polish the enormously precise mirrors that were the heart of the first stage detectors.
A gathering of minds
The Australian Consortium gathered at Gingin earlier this year to plan a new national project.
Part of that project focusses on an 80 meter scale laser research facility – a sort of mini gravity wave detector – the consortium has developed at the site. Experiments are looking at the physics of the new detectors and especially the forces exerted by laser light.
The team has discovered several new phenomena including one that involves laser photons bouncing off particles of sound called phonons. This phenomenon turns out to be very useful as it allows new diagnostic tools to prevent instabilities in the new detectors.
The light forces can also be used to make “optical rods” – think of a Star Wars light sabre! These devices can capture more gravitational wave energy – opening up a whole range of future possibilities from useful gadgets to new gravitational wave detectors.
Final stages of discovery
The first stage detectors achieved their target sensitivity in 2006 and, as expected, they detected no signals. You would know if they had!
The second stage detectors are expected to begin operating next year. The Australian team is readying itself because the new detectors change the whole game.
For the first time we have firm predictions: both the strength and the number of signals. No longer are we hoping for rare and unknown events.
We will be monitoring a significant volume of the universe and for the first time we can be confident that we will “listen” to the coalescence of binary neutron star systems and the formation of black holes.
Once these detectors reach full sensitivity we should hear signals almost once a week. Exactly when we will reach this point, no one knows. We have to learn how to operate the vast and complex machines.
If you want to place bets on the date of first detection of some gravity wave then some physicists would bet on 2016, probably the majority would bet 2017. A few pessimists would say that we will discover unexpected problems that might take a few years to solve.
David Blair receives funding from the Australian Research Council, is a member of the Australian Consortium for Gravitational Astronomy and is a member of the LIGO Scientific Collaboration.
This article was originally published on The Conversation. Read the original article. The views expressed are those of the author and do not necessarily reflect the views of the publisher. This version of the article was originally published on Live Science.