Why Does Time Flow Forward?
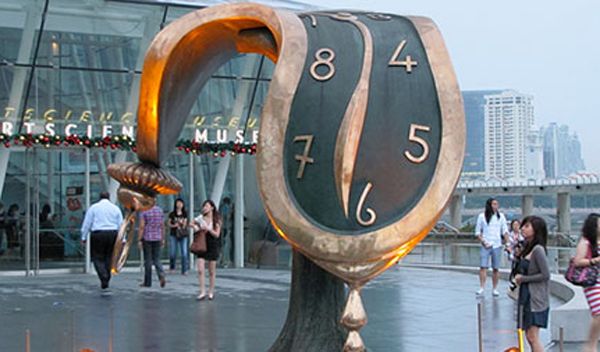
(ISNS) -- Almost nothing is more obvious than the fact that time flows from the past, which we remember, toward the future, which we don’t. Scientists and philosophers call this the psychological arrow of time. Hot coffee left on your desk cools down, and never heats up on its own, which reflects the thermodynamic arrow of time.
In a paper scheduled to appear this week in the journal Physical Review E, two physicists make the case that these two long-separate notions of time — one based on psychology and one based on thermodynamics — must always align.
The principles of thermodynamics show that large collections of particles, like the trillions upon trillions of liquid molecules in a coffee cup, always move toward more disorganized arrangements. For instance, hot water molecules clumped together in a cold room need a lot of organization, so warm drinks eventually cool to the surrounding temperature. Physicists say such disorganized arrangements have high entropy, whereas ordered arrangements have low entropy.
Yet the equations physicists use to describe the simultaneous motions of large numbers of particles are equally valid whether time runs forward or backward. Therefore, almost any complex arrangement of matter will gain entropy no matter which direction time flows.
Our universe apparently began with the Big Bang, which was a special, low-entropy arrangement. This seemingly unlikely and as-yet-unexplained observation gives rise to the thermodynamic arrow of time, which leads cosmologists to observe that the universe is evolving from a lower-entropy past to a higher-entropy future.
Both arrows of time are so intuitive that their distinction is hard to notice, said Todd Brun, a physicist at the University of Southern California, in Los Angeles. “It took the human race millennia before people even realized there was something to be explained.”
In the past century, however, physicists and philosophers have begun trying to unite the thermodynamic and psychological arrows. Many researchers note that real-world objects that store memories -- such as human brains and computer hard drives -- often heat up as they operate. Heat generation increases entropy and is an irreversible process, so the laws of thermodynamics require that such objects can only run in one direction: from past to future.
Sign up for the Live Science daily newsletter now
Get the world’s most fascinating discoveries delivered straight to your inbox.
But memories don’t have to generate heat, point out Brun and physicist Leonard Mlodinow of the California Institute of Technology. For example, ripples on a pond record a rock falling into the water, and yet could, in principle, travel in reverse. Could such a memory remember the future instead of the past, the researchers wondered?
To answer this question, Mlodinow and Brun conducted what is known as a thought experiment. They imagined a system in which a chamber full of bouncing particles is connected to a nearly empty chamber of the same size by a tunnel through which particles can pass one at a time — a low-entropy configuration similar to the concentration of matter in the early universe. Although particles can cross from either chamber to the other, thermodynamics ensures that eventually both chambers will contain roughly equal numbers of particles.
If a rotor is set up to turn each time a particle passes through the tunnel, and every turn of the rotor is recorded, this record would show the arrangement of particles at any time in the past. But according to the laws of motion, the particles’ future locations are completely determined by their current trajectories, and if at any point the flow of time were reversed, the particles would return to their initial low-entropy configuration. So the authors write that the rotor could be seen as recording the system’s future as well — a point made over 200 years ago by the French mathematician Pierre-Simon Laplace.
But there is a catch, Mlodinow and Brun argue. If one were to tweak a future state of the system just slightly, by altering the position or velocity of one or more particles, and then run it backward, the system would no longer decrease in entropy. It would increase.
The particles would begin moving just like a movie playing in reverse, but in anything but the simplest systems, the tweaked particles would soon collide with others and cause a chain reaction. Any snapshot of the system would soon no longer resemble the corresponding frame of the original movie.
Only the exact arrangement of particles resulting from a low-entropy initial state can evolve backward in time to reach a state with such a high degree of order, the authors write. Even minor rearrangements of that system, no matter how similar, will evolve backward toward higher entropy instead. In other words, for any initial condition, the rotor will be able to “remember” only one future that does not violate the thermodynamic arrow of time.
This specificity contradicts the definition of a memory, the authors argue. They write that a memory should be able to include all the ways a system can evolve, not just a particular one. For example, your brain will be able to record virtually any series of events that befall you in the next three hours. If it were capable of recording only one particular series of events, like a speedometer stuck at one speed, your brain would not be a functioning memory. Because of this requirement, even a simple, reversible memory can record only the thermodynamic past and not the future, Mlodinow and Brun write.
With their thought experiment, the authors have created a novel and useful definition of a memory, said Craig Callender, a philosopher of physics at the University of California, San Diego. “It’s new in the sense that they are…really building in a condition to what a memory is.”
Lorenzo Maccone of the University of Pavia in Italy agreed that the authors' research raises an important point by arguing that even a reversible memory should have an arrow of time. But he found that the authors' thought experiment did not describe exactly how the rotor would record the future configurations of particles. Without such a description, Maccone said, “I’m not entirely convinced of [their] explanation.”
Andreas Albrecht, a cosmologist at the University of California, Davis, wishes the authors had questioned the assumptions behind the thermodynamic arrow of time. In a paper recently posted to arxiv.org, Albrecht argued that there may be ways to get a thermodynamic arrow without requiring all the universe’s matter to start in one highly unusual arrangement. By taking this arrangement as a given, Mlodinow and Brun miss an opportunity to explore other possible starting configurations, Albrecht said. “[The study] brushes up so close to incredibly interesting [cosmological] questions and then just walks past them.”
Inside Science News Service is supported by the American Institute of Physics. Gabriel Popkin (@gabrielpopkin) is a freelance science and environmental writer based in the Washington, D.C. area. He has written for Science News, ScienceNOW, Johns Hopkins Magazine and other publications.