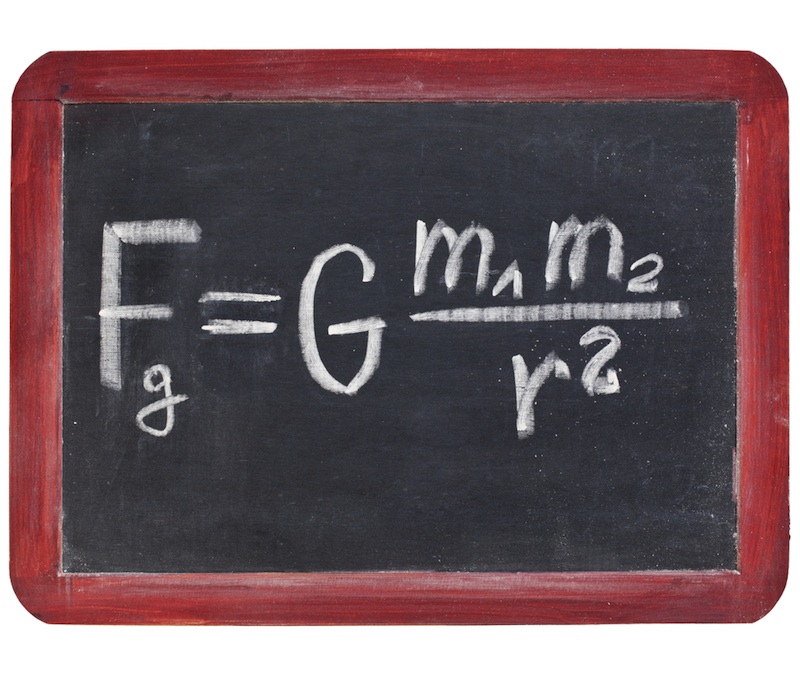
A fundamental constant that sets the size of the gravitational force between all objects has finally been pinned down using the quirky quantum behavior of tiny atoms.
The new results could help set the official value of the gravitational constant, and may even help scientists find evidence of extra space-time dimensions, said study co-author Guglielmo Tino, an atomic physicist at the University of Florence in Italy. [Twisted Physics: 7 Mind-Blowing Findings]
Elusive value
According to legend, Sir Isaac Newton first formulated his theory of gravity after watching a falling apple. In Newton's equations, the force of gravity grows with the mass of two objects in question, and the force gets weaker the more distant the objects are from each other. The English polymath knew the objects' masses had to be multiplied by a constant, or "big G," in order to arrive at the gravitational force between those two objects, but he wasn't able to calculate its value. ("Big G" is different from "little g," which is the local gravitational acceleration on Earth.)
In 1798, scientist Henry Cavendish calculated big G in order to determine Earth's mass. To do so, Cavendish suspended dumbbells on a wire, with enormous lead spheres placed at different distances nearby, and then measured how much the dumbbells rotated in response to the attractive pull of gravity from the neighboring dumbbell. [6 Weird Facts About Gravity]
Since then, almost every attempt to measure big G has used some variation of Cavendish's method. Many of those experiments got fairly precise values — which didn't agree with one another. That's because it was too difficult to identify all potential sources of error in the complicated systems used, said Holger Müller, an atomic physicist at the University of California, Berkeley, who was not involved in the new study.
"The gravitational force is just super tiny, so anything from air currents to electric charges can give you a false result," Müller told Live Science.
Sign up for the Live Science daily newsletter now
Get the world’s most fascinating discoveries delivered straight to your inbox.
As a result, big G is known with much less precision than other fundamental constants, such as the speed of light or the mass of an electron, Tino told Live Science.
Keeping cool
The big systems didn't seem to be working, so the researchers decided to go very small.
The team cooled rubidium atoms to just above the temperature of absolute zero (minus 459.67 degrees Fahrenheit, or minus 273.15 degrees Celsius), where atoms hardly move at all. The researchers then launched the atoms upward inside a vacuum tube and let them fall, in what's called an atomic fountain.
They also placed several hundred pounds of tungsten nearby.
To see how the tungsten distorted the gravitational field, they turned to quantum mechanics, the bizarre rules that govern subatomic particles. At small scales, particles such as atoms can also behave like waves — meaning they can take two different paths at the same time. So the team split the paths the rubidium atoms took as they fell, and then used a device called an atomic interferometer to measure how the waveforms of those paths shifted. The shift in the peaks and valleys of the paths when they recombined was a result of the gravitational pull of the tungsten masses.
The new measurement of G — 6.67191(99) X 10 ^ -11 meters cubed / kilograms seconds ^2 — isn't as precise as the best measures, but because it uses single atoms, scientists can be more confident the results aren't skewed by hidden errors that foiled the more complicated setups of past experiments, Tino told Live Science.
The achievement is impressive, Müller said.
"I thought this experiment would be close to impossible, because the influence of those masses [on gravitational pull] is just very small," Müller told Live Science. "It's really a great breakthrough."
New value
The new experiment raises the hope that future measurements can finally settle on a more precise value for big G.
The findings also could help scientists discover if something more bizarre is at play. Some theories suggest that extra dimensions could warp the gravitational fields in our own four-dimensional world. These distortions would likely be very subtle and would only be noticeable at very small distances. In fact, others have suggested that the different results other labs have gotten were caused by this extradimensional intrusion, Tino said.
By ruling out methodological errors, the new technique could be used to find evidence of extra dimensions, he said.
The new value of G was published today (June 18) in the journal Nature.
Follow Tia Ghose on Twitter and Google+. Follow Live Science @livescience, Facebook & Google+. Original article on Live Science.
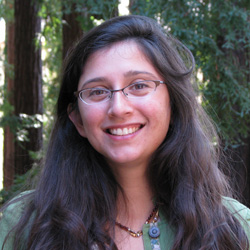
Tia is the managing editor and was previously a senior writer for Live Science. Her work has appeared in Scientific American, Wired.com and other outlets. She holds a master's degree in bioengineering from the University of Washington, a graduate certificate in science writing from UC Santa Cruz and a bachelor's degree in mechanical engineering from the University of Texas at Austin. Tia was part of a team at the Milwaukee Journal Sentinel that published the Empty Cradles series on preterm births, which won multiple awards, including the 2012 Casey Medal for Meritorious Journalism.