Making Headway in Search for Zero-Resistance Wonder Materials
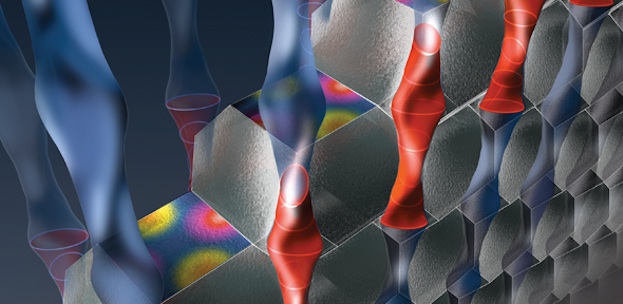
The mystery of how high-temperature superconductors work has endured for 30 years, but a new study could help unravel it.
These materials, which have zero electrical resistance below a certain temperature, have a wide range of applications, from making more efficient electrical grids to creating better supercomputers and magnetically levitated trains.
A team of scientists measured the electronic structure of high-temperature superconductors in a nonsuperconductive state and found that waves of electric charge produce twisted pockets of electrons, which pair up to yield superconductivity. [The 9 Biggest Unsolved Mysteries in Physics]
The findings, detailed June 15 in the journal Nature, could point the way to discovering new superconductive materials, researchers say.
Supermaterials
Most materials are either conductors of electricity or insulators. Conductors usually have some resistance, so some of the electric current gets dissipated (usually as heat). But superconductors are the "perfect" conductors.
"If you took a ring of superconducting material, current would keep flowing throughout the age of the universe," said study researcher Suchitra Sebastian, an applied physicist at the University of Cambridge, in England.
Get the world’s most fascinating discoveries delivered straight to your inbox.
Researchers discovered the first superconductors about 100 years ago, by cooling metals such as copper, mercury and lead below minus 406 degrees Fahrenheit (minus 243 degrees Celsius), near absolute zero. This requires liquid helium, which isn't practical to obtain. Then, about 30 years ago, scientists discovered so-called high-temperature superconductors — copper oxides — that function at only minus 211 degrees F (minus 135 degrees C).
In a normal metal, electrons travel on their own and bump into each other, but in a superconductor, they travel in pairs that allow them to flow smoothly, without losing energy. "It's like having lanes in traffic," Sebastian said.
Scientists already know that in low-temperature superconductors, the crystal structure of the material is what "glues" the electrons into pairs. But what holds the electrons together in high-temperature superconductors has been a mystery, which has made it difficult to know where to find similar materials, she said.
Twisted pockets
In order to understand what makes materials superconductive, Sebastian works with cuprates — thin sheets of copper and oxygen separated by other types of atoms.
"First, I try to kill their superconductivity," she said. Some people do this by heating the material above its superconducting temperature, which breaks up the electron pairs. This temperature change can have unintended effects, however, so instead, Sebastian used a strong magnetic field about a million times the strength of Earth's magnetic field, which banishes superconductivity by breaking the electron pairs.
While the material was in this nonsuperconducting state, Sebastian and her colleagues measured changes in resistance, known as quantum oscillations, which reveal the structure of the electrons.
Previous research suggested that electrons formed "pockets" in the most strongly superconductive regions, but instead, Sebastian found that electrons form twisted pockets in the locations where superconductivity is weakest.
Waves of charge
Sebastian also found that waves of electrons, known as charge order, are what produce these pockets of electrons and result in the material's superconductivity. Just as a magnet is composed of particles aligned by a form of momentum called spin, a material with charge order contains particles that are aligned by positive or negative charge.
Knowing that the normal state of a high-temperature superconductor contains these waves of charge could provide scientists with clues for where to look for other superconductors, Sebastian said.
Other studies have examined these materials in their superconducting state, to understand why electrons sometimes flow smoothly and other times get jammed up. But these studies didn't show how the material's normal structure became superconducting.
"Given that our experiment directly sees the impact of charge order on the electronic structure, and that the electronic structure is closely related to superconductivity — all the complementary experiments come together in a very strong way," Sebastian said.
Follow Tanya Lewis on Twitter and Google+. Follow us @livescience, Facebook & Google+. Original article on Live Science.
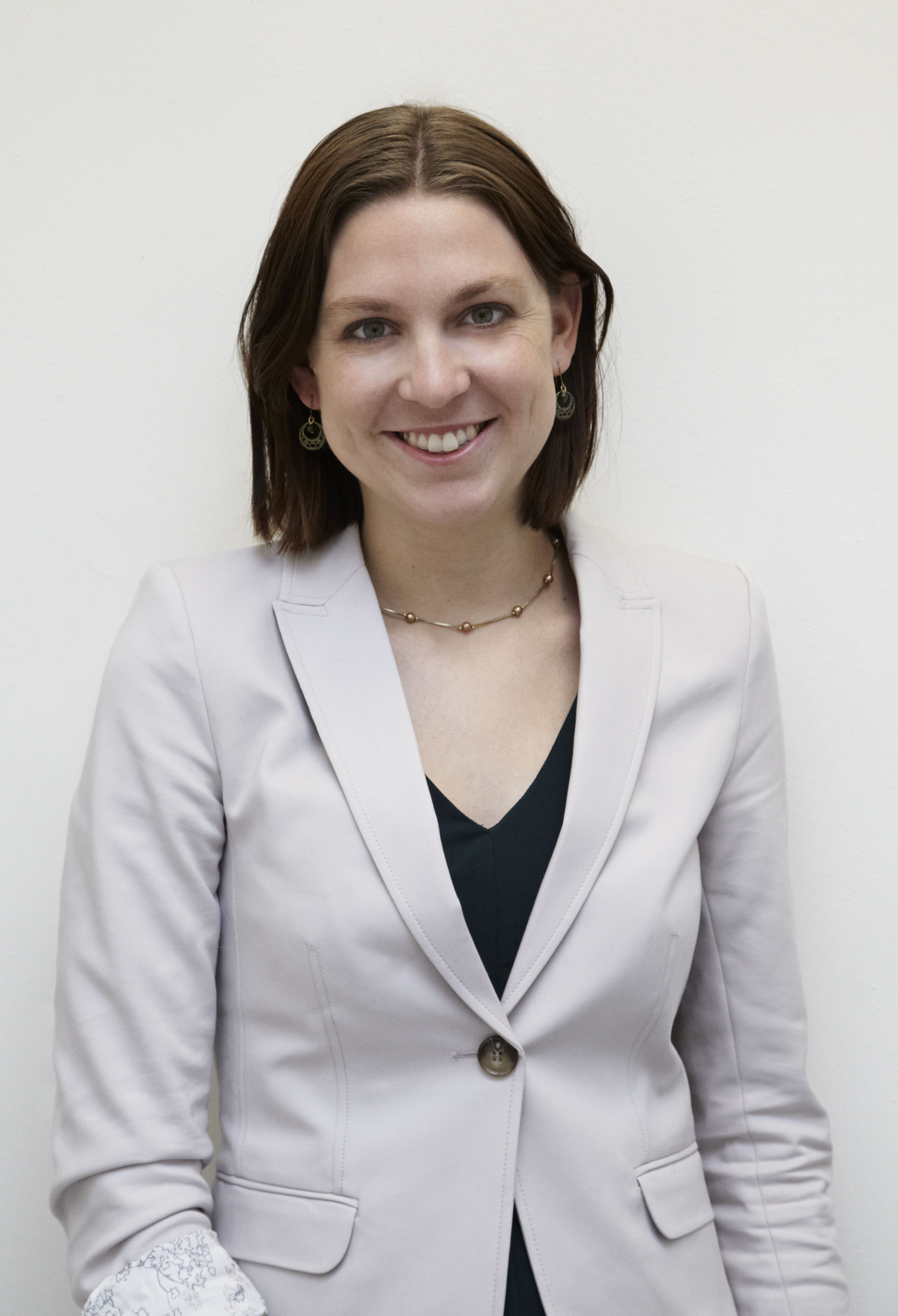