Quantum Experiment Helps Prove Einstein's Theory of Relativity
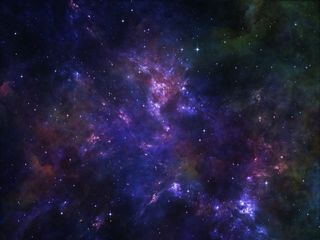
Building a quantum computer can sometimes yield unexpected benefits — like providing the right environment to demonstrate that Albert Einstein's theory of special relativity is, in fact, correct.
Using atoms in certain quantum states, researchers at the University of California, Berkeley, were able to show that space does not appear squeezed in one direction compared to another, as it would if relativity were not correct. Rather, space looks the same from any direction, as relativity predicts. The experiment used partially entangled atoms that were a byproduct of an attempt to build quantum computers.
Special relativity is a cornerstone of modern physics, and was formulated by Einstein in 1905. The theory states two things: the laws of physics are the same everywhere, and the speed of light is a constant, provided that you're not accelerating when you're measuring such phenomena. It can be used to explain the behavior of objects in space and time. (It's companion, the general relativity includes the effects of gravity and acceleration). [Twisted Physics: 7 Mind-Blowing Findings]
Since relativity says the speed of light in a vacuum is constant, space should look the same in every direction, no matter what. For instance, if you move at half the speed of light toward or away from a flashlight, you will see the beam always move at about 186,000 miles per second, no more or less. The concept of time dilation, in which time slows down the faster you go (for example, if you are in a speeding spaceship), is a direct consequence of this phenomenon — it's something that has to happen in order for the speed of light to look the same to everyone in the universe.
Early experiments measuring the speed of light used perpendicular light beams to generate interference patterns — alternating bands of light and dark. Most famous is the Michelson-Morely experiment in 1887, which bounced two light beams between mirrors and showed the speed of light was constant – there was no change in the interference pattern no matter how the apparatus was oriented, which showed there is no "ether" for light waves to pass through, and thus no preferred direction in space. Light speed in a vacuum has one value and one only.
The new study, researchers led by Hartmut Häffner, an assistant professor of physics at UC Berkeley, used atoms. The scientists put two calcium atoms in a vacuum chamber and applied an alternating voltage, which trapped the atoms in place.
Each of the atoms had two electrons, whose energies could be measured. The electrons moved perpendicularly to each other. One in an up-and-down motion, tracing out a volume that looked like a bowling pin around the nucleus, while the other revolved around the nucleus in a toruslike region. In the experiment, the team measured the kinetic energy of the electrons 10 times every second, for a day. If the theory of relativity is correct, then the difference between the electrons' energies should be a constant. [Images: The World's Most Beautiful Equations]
Sign up for the Live Science daily newsletter now
Get the world’s most fascinating discoveries delivered straight to your inbox.
This may seem like a strange way to test a well-established theory, but Häffner said experiments like this have been done with other particles. Electrons, however, give more precise results, he said.
The findings are also important for other areas of physics, including the Standard Model, the reigning theory of particle physics, which describes how particles behave and why the universe appears the way that it does. "The Standard Model depends heavily on special relativity to be correct," Häffner said.
The study also demonstrates how different areas of science are connected, since the experiment started with quantum computing. To make a quantum computer, you need to trap atoms and put them in a special quantum state called superposition. This means that you haven't measured what state the atoms are in, so they can be in two states at once. According to quantum mechanics, until an atom's state is measured, it has no definite value. This is what gives quantum computers their power to solve complex problems much faster than traditional computers can.
It was quantum computing that inspired Häffner to use atoms in such a dual state to test the theory of relativity, he said.
Researchers can use this type of experiment to probe other mysteries in physics and cosmology, the researchers said. For instance, "we can use it to look for dark matter," Häffner said. If there is a lot of dark matter surrounding Earth, the relative energies of the electrons would change, because the presence of the dark matter's mass would alter the surrounding space, he said.
Follow Live Science on Twitter @livescience. We're also on Facebook & Google+. Original article on Live Science.