Tiny 'Crystal Cushion' Drives Earthquakes
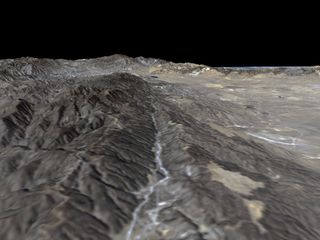
Earthquakes are some of the largest-scale and most-destructive events on the planet, involving plates of the Earth's crust hundreds of miles across. But new research shows that the physics of Lilliputians govern this shuddering of giants.
Researchers found that both earthquakes that occur close to the surface and deeper tremors involve the same culprit: a lubricant made of nanometer-size crystals. The crystals form when rock is heated and subjected to pressures so high the material actually changes its state, much as water will turn into exotic forms of ice if the pressure is high enough. In both deep and shallow earthquakes, the nanocrystals free the slabs of rock to slide past each other and trigger a quake.
"Both deep and shallow quakes end up sliding on a nanocrystal cushion," Harry W. Green II, a professor of earth sciences at the University of California, Riverside, told Live Science. "They just get there by different paths." [Image Gallery: This Millennium's Destructive Earthquakes]
Nanocrystal lubricant
Using computer simulations, Green and his colleagues found that earthquakes at shallow depths, less than 18 miles (30 kilometers) down, involve mechanics just like those occurring between 180 and 430 miles (300 and 700 km) down.
Earthquakes tend to happen at the boundaries of tectonic plates. In California, the North American plate slides against the Pacific plate to trigger earthquakes along the San Andreas Fault. In Nepal, the Indian plate crashes into the Eurasian plate, pushing up the Himalayas, before forcing its way underneath the Tibetan plateau. Such sliding leads to earthquakes as the plates catch, and then suddenly slip. Those quakes' origins tend to be within a dozen or so miles of the surface.
For deeper tremors, things happen a little differently, as these quakes occur at the top of the mantle and often near subduction zones, where one plate is sliding beneath another. Such earthquakes occurred in the Sea of Okhotsk in 2013 and Bolivia in 1994. Despite both being magnitude-8 temblors, neither caused much damage as they were far from the surface.
Sign up for the Live Science daily newsletter now
Get the world’s most fascinating discoveries delivered straight to your inbox.
To understand what happens during shallow earthquakes, the scientists looked at rock from a fault that is an ancestor to the San Andreas, called the Punchbowl, also in California. Samples of the rock showed nanometer-size crystals embedded in pieces of a mineral called feldspar. The crystals formed due to the enormous heat and pressure on the rock.
This was close to what Green and some other geologists had predicted they might find. But there was another mystery that has dogged geologists for decades: Why doesn't the San Andreas Fault produce more heat? And why is glass so rare near faults? All that frictional heat and pressure should ramp up temperatures along the fault, and in some cases melt the local rockinto glass. But that isn't what geologists see. Instead, they find that glass is rare and the fault doesn't look any hotter than surrounding rock.
The solution was the chemical reaction that creates the nanocrystals: it is endothermic, meaning it absorbs heat. All that heat energy that would have been radiated both during quiescent periods, when the rocks are slowly grinding against each other, and during an earthquake, when they slip and slide, was taken up by the chemical reaction, which only happens at high pressure and temperature.
Meanwhile, for a number of years, Green and other scientists have been testing the effects of high pressures on materials thought to be similar to those found in deep subduction zones. The result is similar: nanometer-size particles.
This time, the particles are made in a kind of runaway chain reaction, one that releases heat rather than absorbing it. The released heat powers chemical changes that make more crystals. The crystals act as a lubricant that lets the rocks slip by each other. This explains a lot because at those depths many models predicted there had to be some kind of fluid allowing the rocks to move. The nanocrystal model shows fluid isn't necessary.
The study is detailed in the May 18 issue of the journal Nature Geoscience.
Follow Live Science @livescience, Facebook & Google+. Originally published on Live Science.