'Impossible' Feat: Scientists Measure Energy of Atoms During Reactions
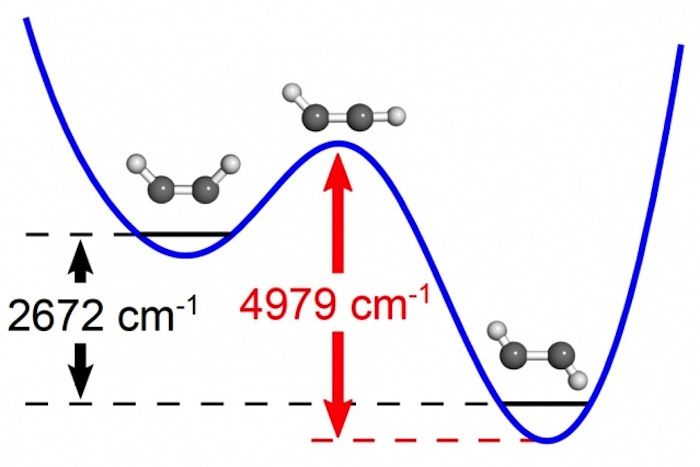
For the first time, scientists have accomplished a feat long thought impossible — they have measured the energy of incredibly short-lived arrangements of atoms that occur as chemical reactions are happening.
This finding could help shed light on the precise inner workings of chemical reactions too complex to understand by other methods, the researchers said.
The chemical reactions responsible for life, death and everything in between involve molecules transforming from one kind to another — essentially, from reactants to products. As chemical reactions occur, fleeting and unstable arrangements of atoms, known as transition states, exist as molecular bonds break and form between atoms. [Wacky Physics: The Coolest Little Particles in Nature]
"Reactants and products are stable valleys on either side of a mountain range, and the transition state is the pass," study lead author Joshua Baraban, a physical chemist at the University of Colorado Boulder, said in a statement. "Because it only exists as you go from one thing to another, it's never really been thought of as something that you can easily study directly."
Now, for the first time, scientists have measured the amount of energy required to reach a transition state.
"This is something that, if you asked people with a Ph.D. in chemistry, they'd say it was not possible to do," Baraban told Live Science. "There are textbooks that say this is not possible to do."
The researchers investigated a kind of chemical reaction known as an isomerization, in which a molecule undergoes a change of structure. They focused on a molecule known as acetylene, which consists of two carbon atoms and two hydrogen atoms.
Sign up for the Live Science daily newsletter now
Get the world’s most fascinating discoveries delivered straight to your inbox.
When acetylene absorbs energy, there are two conformations it can adopt, which can be visualized by imagining the atoms as balls and the molecular bonds connecting the atoms as sticks. In acetylene, the carbon atoms are bound to each other and make up the middle of the molecule, and each carbon atom has one hydrogen atom attached to it.
One conformation has a zigzag shape, in which one hydrogen atom is positioned on one side of the carbon-carbon bond, while the other is on the other side of the carbon-carbon bond. The other conformation is shaped like a "U," with both hydrogen atoms on the same side of the carbon-carbon bond.
With a bit of energy, the zigzag version of acetylene can become the U-shaped kind, the researchers said. In between, a transitional state occurs where one of the hydrogen atoms is not positioned on either side of the carbon-carbon bond, but instead is almost in line with it.
The researchers used lasers to monitor changes in acetylene vibrations as the researchers gave more energy to the molecules. When specific levels of energy were reached, the patterns of vibrations changed in the kinds of ways expected near the transition state, the researchers said.
This kind of change in conformation is also an important part of how the eye works. "When light enters the eye, it causes this kind of change we see in acetylene, which starts a chain reaction that sends information that the eye has seen a photon to the brain," Baraban said.
The scientists also showed that they can use their technique to accurately predict the structure and energy of the transition state between hydrogen cyanide and hydrogen isocyanide. In hydrogen cyanide, a hydrogen atom is connected to a carbon atom, which, in turn, is bound to a nitrogen atom. In hydrogen isocyanide, a hydrogen atom is connected to a nitrogen atom, which, in turn, is bound to a carbon atom. The transition state between these molecules has one hydrogen atom, one carbon atom and one nitrogen atom bound to one another like a triangle.
Future research can analyze more complex reactions, such as ones where two molecules come together or one molecule breaks into two, the scientists said.
Baraban, along with study senior author Robert Field at MIT and colleagues, detailed their findings online today (Dec. 10) in the journal Science.
Follow Live Science @livescience, Facebook & Google+. Original article on Live Science.