Tiny Artificial Life: Lab-Made Bacterium Sports Smallest Genome Yet
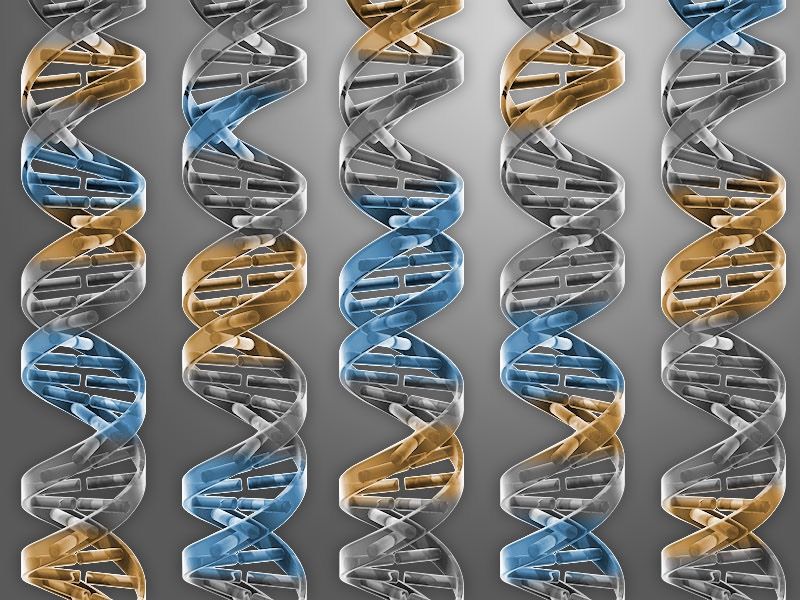
An artificial bacterial genome with the smallest number of genes needed for life has been created in a lab, opening the way for creating synthetic organisms with customized sets of genes aimed at specific tasks, such as eating oil.
The newly created bacterium, which can metabolize nutrients and self-replicate (divide and reproduce), brings the team one step closer to building custom artificial life with particular functionalities, they said.
The artificial bacterium has only 473 genes, compared with the thousands that exist in wild bacteria. The team doesn't yet know the function of 149 of these essential-to-life genes. [Unraveling the Human Genome: 6 Molecular Milestones]
"We're showing how complex life is even in the simplest of organisms," said Craig Venter, founder and CEO of the J. Craig Venter Institute (JCVI), where the study was completed. "These findings are very humbling in that regard."
The story starts with a genus of bacteria called Mycoplasma, germs that have the smallest genomes of any organism found in nature and tend to live in humans and other mammals.
Venter said he and another of the study's authors, Clyde Hutchison of JCVI, had discussed in the 1990s what it would take to answer basic questions about the way life functions. Their conclusion was that they'd need to build an organism with the smallest genome possible.
In 1995, Venter said, other researchers estimated such an artificial organism would need, at minimum, 256 genes to be viable. That turned out to be wrong – but it wasn't until now that they knew just how wrong.
Sign up for the Live Science daily newsletter now
Get the world’s most fascinating discoveries delivered straight to your inbox.
The team used the genome of the M. mycoides to create their bacteria. That bacterium's genome was synthesized in 2010, creating the first self-replicating cell from an artificial genome. The Venter Institute called that bacterium syn1.0. That bacterium, though, had 1.1 million base pairs in its DNA, or 901 genes.
Their new bacterium has 531,000 base pairs, for 473 genes. To cut down the number of genes the team used the syn1.0 genome as a template. From there they designed a set of possible genomes for the bacterium and broke them into shorter strings. To see which genes were absolutely necessary for life, the scientists inserted genetic sequences called transposons that disrupted the functioning of a given gene. If after that the cell stayed alive, then it was considered nonessential, and snipped out. Conversely, if the cell died, then it was clear that whatever was taken out was essential.
However, the process wasn't as simple as that, Venter said. Sometimes a single gene could be removed by itself, but coupled with another it became essential. Venter likened it to an aircraft: "If you know nothing about airplanes and you're looking at a 777… and you remove the right wing, the airplane can still fly and land, so you'd say it's not essential, and you don't really discover the essentiality until you remove the second one."
Eventually they built a synthetic genome that could be inserted into another Mycoplasma bacteria (the old genome is removed), which on its own was able to grow and live like a normal cell. They called the result syn3.0. [Infographic: How Scientists Created a Semi-Artificial Life Form]
Venter and his team added that the minimum number of genes required for life would differ depending on what organism they started with — they would get a very different result had they begun with an algae species, for example. Which genes are essential can also depend on the environment a cell or bacterium is in.
For example, in early work on Mycoplasma genitalium, the growth media was both fructose and glucose. Knocking out a gene that transports fructose may not affect a cell that is in a glucose-rich environment, and knocking out a glucose transporter wouldn't affect it, either. But if both are knocked out, then the cell will die. So which gene is essential is not an all-or-nothing proposition.
Hutchison, lead author of the study and a distinguished investigator at JCVI, noted that the minimal genome would also depend on what one wants the cell to do — a bacterium that glows in the dark will have a different minimal genome than something else.
"There will be lots of minimal genomes," Venter said.
Maria Lluch Senar, a staff scientist and biotechnologist at the Center for Genomic Regulation in Barcelona, Spain, said the achievement is exciting, because it has revealed a method for designing genomes that is much faster than the trial-and-error methods currently used. "The thing is here you can identify which is the minimal genome you want," she said, for a given function. "With this technique you can define which is the best combination of fragments of DNA … You can assemble them later on and generate different molecules that can be tested."
"In theory, we could add gene sets and essentially recreate any organism," Venter said. "It would be an important experimental tool."
That said, the technique promises better avenues for making germs that do everything from eating oil to making biofuels.
"Our long-term vision has been to design and build synthetic organisms on demand where you can add in specific functions and predict what the outcome is going to be," said study co-author Dan Gibson, an associate professor at the Venter Institute.
A minimal cell would devote the maximum amount of energy into whatever you designed the cell to do, and have less potential to mutate, and be easier to engineer, Gibson said.
That ability to add gene sets could also aid in the understanding of why some bacteria evolved the way they did — and even life in general, though that's more of a stretch, Hutchison said. "We may be seeing some processes that occurred early in evolution," he said. "But [Mycoplasma's genomes] are not small because they are primitive, they are small because they evolved from a cell that had a few thousand genes and they've lost genes that they don't need in their environment."
Venter said the plan is to keep working on adding genes to the synthetic genome, to tease out the functions of the unknown genes. "We want to get to where we understand 100 percent of the genes in the organism, not just 66 percent."