
Dark Matter Just Got Murkier
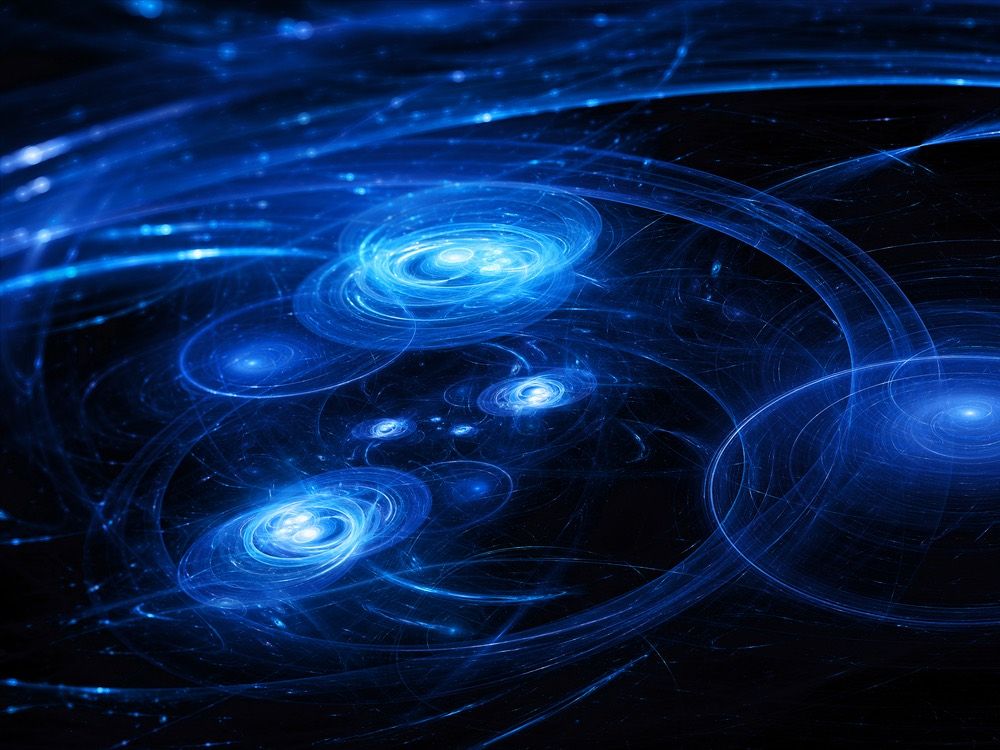
Don Lincoln is a senior scientist at the U.S. Department of Energy's Fermilab, America's largest Large Hadron Collider research institution. He also writes about science for the public, including his recent "The Large Hadron Collider: The Extraordinary Story of the Higgs Boson and Other Things That Will Blow Your Mind" (Johns Hopkins University Press, 2014). You can follow him onFacebook. Lincoln contributed this article to Live Science's Expert Voices: Op-Ed & Insights.
They say that love makes the world go around and that may well be true. But when you look at things on a much larger scale — say the size of galaxies — love just isn't enough. And, for that matter, neither are the stars of the galaxies themselves. In fact, what makes galaxies go around is a kind of matter that has never been directly observed. That undiscovered "stuff" is called dark matter, and an amazing new measurement was recently announced that is causing the scientific world to rethink long-held thoughts.
The most recent contribution to our knowledge of dark matter was made by the Large Underground Xenon (LUX) collaboration. LUX is a vessel consisting of a third of a ton of liquid xenon and it is the most powerful dark-matter detector ever constructed. Located at the Sanford Underground Research Facility (SURF), this dark-matter laboratory sits nearly a mile under the Black Hills, near Lead, South Dakota. It is designed to occasionally detect the vaporous wind of dark matter that is thought to waft through the solar system. [6 Cool Underground Science Labs]
And the thing is, it didn't detect anything. That non-discovery is what's causing physicists to rethink how they think about dark matter.
Holding up galaxies
Dark matter is an answer to a nearly century-old problem. In the early 1930s, shortly after astronomers realized that the universe consisted of countless galaxies, scientists turned their attention to understanding the dynamics of how stars orbited within the galaxies — essentially, how galaxies rotate. Dutch astronomer Jan Oort applied Newton's laws of motion and gravity to the observed matter in our own Milky Way and found that our galaxy rotated faster than he had calculated. It appeared that the Milky Way had twice the mass that astronomers had estimated. Of course, this was in an era in which precise galactic astronomy was coming into existence and a disagreement between calculation and measurement of only a factor of two was considered excellent agreement.
However, shortly after Oort's measurement, Bulgarian-Swiss astronomer Fritz Zwicky was studying the Coma Cluster, a large group of more than a thousand galaxies that were bound together by their mutual gravity in an enormous structure. When he measured the velocity of the galaxies, he found that they, too, were moving far too fast for gravity's gentle tug to hold them together. By all rights, the cluster should have torn itself apart. But it didn't. He calculated that the cluster held 400 times more matter than could be seen by ordinary telescopes. Modern measurements have reduced that number, but the discrepancy still is thought to be a factor of 100. Zwicky proposed that there was a type of unseen matter holding together the cluster that he called "dunkle Materie," or dark matter. [TED-Ex Talk: How Does Dark Matter Explain a Star's Speed?]
In the 1970s, astronomer Vera Rubin was trying to find a noncontroversial topic to study when she turned her attention to the rotation curves of galaxies. This is a measure of the orbital speed of stars in galaxies as a function of their distance from the center. She found that the measurements agreed with predictions very well at the center of the galaxy and even approaching the periphery. But, in the very outskirts of the galaxy, stars were orbiting far faster than could be accommodated by the known laws of physics and the observed matter. It looked like Zwicky's dark matter might be showing up in the interiors of galaxies as well. (Clearly, Rubin failed epically in her attempt to find a noncontroversial topic.)
Sign up for the Live Science daily newsletter now
Get the world’s most fascinating discoveries delivered straight to your inbox.
Over the decades, several ideas have been proposed to explain an extensive array of astronomical mysteries, from the possibility that Newton's laws of motion might not apply when accelerations become small, to the idea that both Newton and Einstein were wrong about gravity. These hypotheses have not survived rigorous testing. Another idea was that perhaps there exist types of matter in the universe that do not emit electromagnetic energy … this was Zwicky's dark matter.
But, even here, there were many possibilities. The most plausible option was that the universe was home to a menagerie of black holes, brown dwarfs, rogue planets and other dark objects that consisted of the same kind of ordinary matter that makes up the visible component of the universe. These objects are massive and compact and cold enough that they don’t emit light like stars. Some objects like these were found, but not enough to solve the mystery. And so, astronomical surveys in the 1990s completely ruled out this idea, too. Taking a page from Sherlock Holmes in “The Sign of Four,” in which he said, “When you have ruled out the impossible, whatever remains, however improbable, must be the truth,” scientists have been forced to conclude that a new form of invisible dark matter permeates the universe. Perhaps even more surprising, there appear to be five times more dark matter than ordinary matter.
The properties of dark matter
We have never directly observed dark matter, but we know a great deal about what it must be: It must be massive (because it affects the rotation of galaxies); it must be electrically neutral (because we can't see it); it must be different from ordinary matter (because we see no evidence for it interacting with matter in the usual ways); and it must be stable (because it has existed since the dawn of the universe). These properties are unequivocal.
However, we don't know exactly what it is. In the most popular generic theory, the dark-matter particle is called a WIMP, for weakly interacting massive particle. WIMPs are kind of like heavy neutrons (but definitely not neutrons), with a mass of 10 to 100 times heavier than a proton. They were created in great quantities during the Big Bang and a small relic remainder persists to this day.
When cosmologists add the WIMP idea to their ideas of the Big Bang, they can calculate how it would interact. They find that in the earliest stages of the universe, WIMPs were a dominant form of matter, but, as the universe expands and cools and the interaction rate drops to zero, the relic amount of WIMPs is about five times as massive as ordinary matter. Combined with the fact that the WIMP idea can also explain a pressing theoretical mystery of why the Higgs boson particle has such a small mass, scientists call this the "WIMP miracle," in view of the fact that the WIMP idea seems to answer so many questions.
A dark non-discovery
So this brings us back to the LUX experiment. It is simply the latest and most powerful experiment designed to detect WIMPs. The idea is that the detector will sit underground for a long time, say a year or more, and a rare WIMP will bump into a xenon atom and be detected. (The detector is buried underground to protect it from cosmic rays, which would bump into xenon atoms and fake a dark matter detection. Being half a mile or a mile underground stops nearly all cosmic rays.)
On Aug. 27, the LUX researchers announced their results. They saw no evidence for dark-matter WIMPs.
So that's not actually that surprising. There have been dozens of experiments that have looked for dark matter and found nothing. In this sense, LUX has joined a venerable group. But LUX is also far more capable. It was expected to have a better shot at finding WIMPs than any other apparatus.
But it didn't.
So does that mean that the WIMP idea is dead? No, not really. Dark matter detectors are optimized to be most sensitive to certain masses, kind of like how a particular microphone might pick up tenor voices better than they hear soprano or bass ones. LUX was optimized to find a WIMP with a mass of a few tens of times heavier than a proton. In fact, it ruled out a WIMP having a mass 50 times of a proton with impressive rejection. However, LUX doesn't do so well at detecting WIMPs with a mass below 10 times that of a proton. And, if WIMPs exist and have a mass of 1,000 times heavier than a proton, this is also not LUX's optimum range for investigation. So there remains a range of masses where a WIMP might exist.
Axions, dark life and dark charge
Even so, WIMPs were simply the most popular idea for dark matter. There are other hypotheses. One hypothesis points to a particle called a sterile neutrino, which is a cousin of the more familiar neutrino generated in nuclear reactions. In fact, neutrinos from the biggest nearby nuclear reactor (the sun) are constantly barraging the Earth. Unlike regular neutrinos, which are ultralight and interact via the weak nuclear force, sterile neutrinos are heavy and don't experience the weak force. It is the heavy mass and non-interacting qualities that make the sterile neutrino an ideal dark-matter candidate.
Another possible dark-matter particle, the axion, was proposed in 1977 as a way to ensure that the strong nuclear force treated matter and antimatter on equal footing (so as agree with observations). The axion is a very light, but still massive, hypothetical particle. The LUX detector is not designed to study axions.
Then, of course, there is the even more creative hypothesis, which suggests that dark matter isn't a single, neutral, non-interacting particle. After all, ordinary matter is pretty complicated. At the quantum scale, we have quarks and leptons and four forces. At the macro scale, we have you and me and sugar and stars and volcanoes and all the various ways they interact. Ordinary matter has all sorts of interactions and constituents. Why not dark matter? [7 Strange Facts About Quarks]
Under this hypothesis, dark matter might have a "dark charge," or dark matter's form of electrical charge. In the same way that dark matter doesn't experience dark charge, maybe ordinary matter doesn't experience dark charge. This dark charge would interact with dark photons, or the dark twins of ordinary light particles.
And, even more daring, perhaps dark matter experiences many forces and there is an entire complex dark sector, with dark atoms and dark molecules and perhaps even dark life. We are able to set constraints on these possible dark interactions; for instance, we know enough to rule out dark stars and planets, but dark asteroids are possible. Again, LUX is silent on these new ideas.
It's not fair to say that the LUX measurement leads to a crisis in particle physics and cosmology. But it certainly gives scientists pause and suggests that maybe we should take another look at this WIMP thing. Maybe other ideas need to be revisited. On the other hand, scientists who want to continue to pursue the WIMP idea still have something to look forward to as technology advances. LUX uses a third of a ton of liquid xenon. In 10 or 15 years, scientists are planning to build detectors that might contain 100 tons, providing even more chances of capturing that rare WIMP interaction. These are heady times to be a dark-matter scientist.
But, in the end, we still don't know. We just know that the capabilities of LUX are good enough that maybe it's time to broaden our thinking. In the words of the rock band Buffalo Springfield, "There's something happening here. What it is ain't exactly clear..."
Follow all of the Expert Voices issues and debates — and become part of the discussion — on Facebook, Twitter and Google+. The views expressed are those of the author and do not necessarily reflect the views of the publisher. This version of the article was originally published on Live Science.