Crushed Crystal Reveals a Spookier Entanglement State
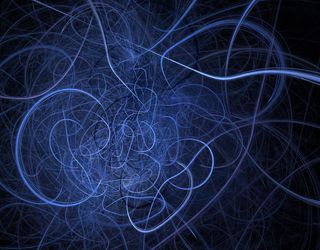
Like pairs of spinning dancers that suddenly form a quartet, the magnetic "spins" of electrons can become entangled in groups of four, new research reveals.
The new quantum state, called a plaquette singlet, solves a longstanding question about quantum mechanics, the mysterious laws that govern the behavior of tiny subatomic particles. The work may also open the way to new kinds of electronics that go beyond the binary logic of 0's and 1's in all modern computers.
In the new research, physicist Mohamed Zayed, a physicist at École Polytechnique Fédérale de Lausanne (EPFL) in Switzerland, and colleagues took a piece of strontium copper borate, a compound similar to high-temperature superconductors, and put it under high pressure while cooled to a few degrees above absolute zero. As they ratcheted up the pressure, they found that the electrons in the material entered a state no one had ever seen before, in which electrons' magnetic spins were entangled with each other in groups of four. Such a state had been predicted, but never actually observed. [The 18 Biggest Unsolved Mysteries in Physics]
Such changes of state (called phase changes) are the basis of modern electronics, Zayed said. For example, semiconductors work because they morph from insulators to conductors at specific voltages, turning circuits "on" and "off." This ability to switch from "on" to "off" creates the 0's and 1's that form the binary logic at the heart of computer calculations.
If harnessing one phase transition – that of an insulator changing to a conductor – leads to binary computer technology, "mastering some of all those other available phase transitions could lead to completely novel technologies hard even to imagine at this stage," Zayed said in an email to Live Science.
High-pressure phenomenon
Scientists already knew how strontium copper borate behaved at low pressures. The material forms a two-dimensional lattice, with all the electrons laid out like a set of square tiles. Each electron has a "spin" – one can imagine them as tiny magnets, with the spin described as "up" or "down." (In fact, spin is just a mathematical way to describe the magnetic fields around the electron and is not really a rotating object).
Electron behavior is governed by quantum mechanics, so the spins can only have discrete values. Further, quantum mechanical particles can be entangled – some properties can be linked so that the particles behave as a single unit. In this case, pairs of electrons' spins are entangled. [Infographic: How Quantum Entanglement Works]
Sign up for the Live Science daily newsletter now
Get the world’s most fascinating discoveries delivered straight to your inbox.
When the pressure goes up, the arrangement of the electrons alters slightly, because the distance between electrons changes. The EPFL team subjected the strontium copper borate to pressures as high as 800,000 pounds per square inch (55,000 atmospheres). At approximately 21,500 atmospheres, something changed: the electrons' spins were entangled in groups of four rather than two — a state called a plaquette singlet.
To "see" the new quantum state, the scientists fired neutrons at the experimental sample; neutrons have zero charge but they do have a magnetic field, and the behavior of the neutrons after they hit the strontium compound revealed the entanglement state of the electrons.
Predicting complex behavior
While that particular quantum state had been predicted before, nobody was sure it would actually happen, said study co-author Henrik Rønnow, a quantum physicist also at EPFL. One reason is the mathematics are difficult to do; it was one of several possibilities.
Theoreticians have calculated the behavior of particles in one-dimensional settings (imagine the electrons in a straight line) and a few two-dimensional ones. But multiparticle 2D systems become more complex.
"Two particles is easy to deal with," Rønnow told Live Science. "But doing those same calculations for more than two particles is hard. "When you get to 20 or 30 particles even the best computers will run out of steam."
A theory called the Shastry-Sutherland model predicts how a 2D lattice of electrons in the strontium compound should behave; it has what are called exact solutions as long as the pressure and temperature are relatively low (meaning less than tens of thousands of atmospheres of pressure and near-absolute zero). The math was less certain under different conditions, hence the experimental tests.
Now that they know what happens, Rønnow said, it's possible to refine theories of how particles behave, especially in solid-state systems. "It opens a field for more study of comparing theory to experiment," he said. "We had maybe ten different theories trying to predict what would happen here. Now theoreticians can go back and say what went wrong."
The study appeared July 17 in the journal Nature Physics.
Originally published on Live Science.