Oddly Behaving Blobs Beneath Earth's Surface Finally Explained
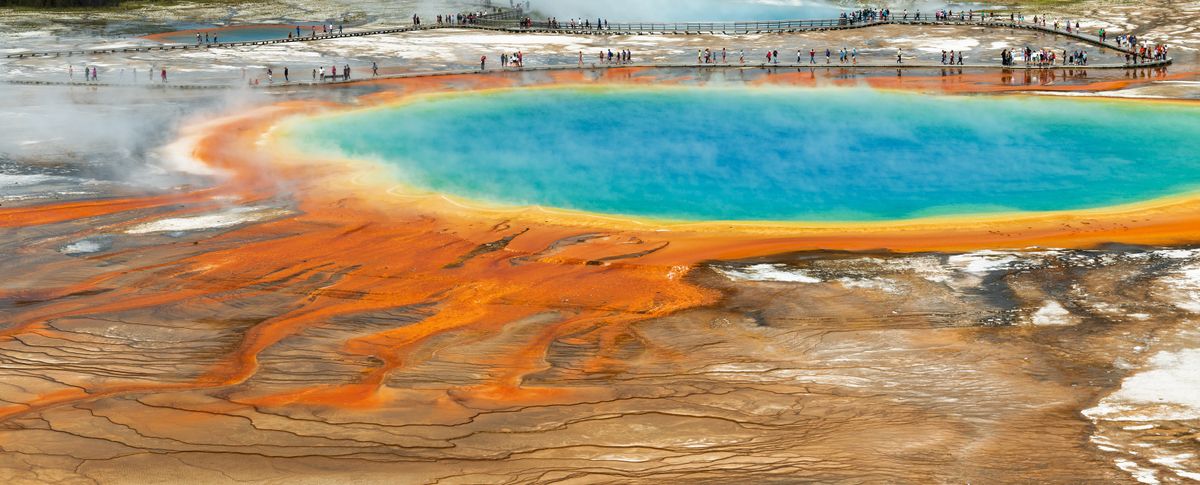
The boundary between the Earth's outermost layer, the crust, and the underlying mantle is speckled with mysterious, blob-like regions. Scientists have long known about these odd pockets, which are called ultralow-velocity zones. They slow down the seismic waves caused by earthquakes and may be the culprit for deep mantle plumes, which can lead to volcanic hotspots like those that created Yellowstone National Park or the Hawai'ian Islands.
Researchers have postulated a number of explanations for what these ultralow-velocity zones are made of and how they're formed. But none of those ideas quite fit the data, especially given how differently some of the zones behave from one another.
Now, a team of scientists is proposing a new model that includes not only a feasible composition but also a plausible origin story for ultralow-velocity zones. Even so, the scientists behind the study concede that there could be different or even individual variations for other types of these mysterious, subterranean regions beyond their new findings. [7 Ways the Earth Changes in the Blink of an Eye]
The core-mantle boundary in which ultralow-velocity zones reside is incredibly hot and pressurized — enough so that when the tectonic plates push beneath the crust via subduction, they are melted down and recycled into the mantle. Pressure levels reach 900,000 times what we experience at sea level and temperatures can exceed 3,000 degrees Fahrenheit (1,649 degrees Celsius).
When the team of geologists from Stanford University re-created these conditions in a lab to try to duplicate an ultralow-velocity zone, they found that iron — one of the more abundant minerals on Earth and a likely candidate material that might explain the zones — reacted with the seawater to create a form of iron peroxide that was saturated with hydrogen atoms, according to the new research.
This additional hydrogen makes the iron peroxide stable under extreme conditions and denser than surrounding minerals, giving rise to the distinct zones that behave differently than the rest of the mantle. The scientists then conducted a theoretical analysis based on the current body of scientific knowledge about Earth's mantle, ultralow-velocity zones and their best assumptions, finding that hydrogenated iron peroxide would interfere with earthquake shockwaves as expected. Without the added hydrogen, that wouldn't be the case.
"The low sound speeds we calculated for our compound match very well what is observed seismically in ULVZs [ultralow-velocity zones]," said Jin Liu, a geologist who worked on the research.
Sign up for the Live Science daily newsletter now
Get the world’s most fascinating discoveries delivered straight to your inbox.
The researchers conducted their experiment on a piece of iron foil submerged in water that was then pressurized and heated with lasers, but they argue that natural conditions beneath Earth's crust can also give rise to the iron peroxide that they think makes up ultralow-velocity zones.
Seawater is regularly forced beneath Earth's crust, along with subducting tectonic plates, where it interacts with an abundance of iron. The scientists calculated that about 2.2 trillion pounds (1 trillion kilograms) of water are pushed into the mantle every year. Most of that returns to the surface via volcanic activity, but about 640 billion pounds (300 billion kg) of water continue into the deeper mantle, where it can react with iron. Assuming this process has been going on for all 4.5 billion years of Earth's existence, the researchers say that all known ultralow-velocity zones could have been formed in this manner, even if just 100 billion pounds (45 billion kg) of water — one-10th of all the oceanic water on Earth — reacted with iron each year.
This newly unearthed mechanism, detailed Nov. 22 in the journal Nature, could give rise to a better understanding of how the planet works deep beneath the surface.
"ULVZs could hint at much greater cycling between the core, mantle and surface of Earth than we thought," said Wendy Mao, the Stanford geologist who led the research team.
Original article on Live Science .