Dying Brains Silence Themselves in a Dark Wave of 'Spreading Depression'
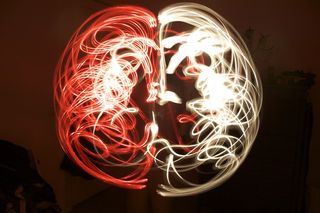
At the edge of life and death is a spreading dark wave.
Scientists spotted it first in rabbits. In a series of papers published throughout the 1940s, Harvard biologist Aristides Leão described finding a sudden silencing of electrical activity in the exposed brains of his unconscious experimental animals after subjecting them to injuries — applying electrical shocks, poking them with glass rods or cutting off the blood in their arteries. The "spreading depression," as he termed it, began at the injured spot within 5 minutes of the injury, before eclipsing more distant parts of the brain.
Seven decades later, a paper published Feb. 15 in the journal Annals of Neurology reveals just how this process occurs in the dying brain cells of humans. [10 Mysteries of the Mind]
To gather their data, the researchers set up shop in hospitals in Berlin and Cincinnati. With consent from next of kin and other legal representatives, they reported results from nine patients who died with electrodes implanted in their brains, recording how their neurons behaved in their last minutes. All of them had existing conditions that required invasive neural monitoring, so the electrodes were already in place when doctors stopped life-preserving care.
The people in the study had severe brain injuries: a "47-year-old male occupant of a car struck by train," a "57-year-old male who was found at the base of a stairway," and heart attack and stroke victims. So they likely had already gone through their first "spreading depression" before the electrodes were applied. This paper describes the final depressions before final brain death.
As the body dies, brain cells sputter electrical juice
Like other organs, brains are made up of flesh, which means they are made up of cells — neurons, mostly. And neurons rely on delicate chemical balances to function. The new paper, the first to study in detail in humans the chemical processes Leão discovered in rabbits, reveals a very similar process preceding final, irreversible death.
Neurons work by filling themselves with charged ions, creating electrical imbalances between themselves and their surroundings that let them generate the little shocks that constitute their signals. And maintaining that imbalance, the authors wrote, is a constant effort — electromagnetic forces tug on the ions, trying to wipe out the charge imbalance even as the neurons labor to maintain it.
Sign up for the Live Science daily newsletter now
Get the world’s most fascinating discoveries delivered straight to your inbox.
To fuel that effort, those hardworking cells drink greedily from the bloodstream, gulping oxygen and chemical energy. When the body dies, and blood flow to the brain stops, oxygen-deprived neurons try to hoard their remaining resources, the researchers wrote.
Sending signals back and forth is wasteful of those precious last sips of life. So, as much as possible, the neurons go silent, instead using their remaining energy stores to maintain their internal charges, waiting for the return of a blood flow that will never come.
To researchers observing with electrodes, this first wave of darkness doesn't seem to spread. Instead, they wrote, it happens everywhere at once, as neurons all over the brain react to the sudden drought. The final, spreading wave comes minutes later, as cells run out of their limited chemical stores, and their stored ions leach into the surrounding tissue — and millions of batteries suddenly lose their charge.
This marks, for dying patients, the final moments of brain function, the authors wrote. But they cautioned that it's an imperfect marker of true death. Previous research in animals has shown that if blood and oxygen return quickly enough to the brain after the spreading wave, the neurons can return to life and recover their chemical charge. Only after sitting in their depolarized chemical soup for a few minutes after the spreading wave, it seems, do neurons reach a somewhat vague "commitment point" beyond which there is no return.
Originally published on Live Science.