Researchers Just Measured an Atom with a Half-Life of 18 Sextillion Years
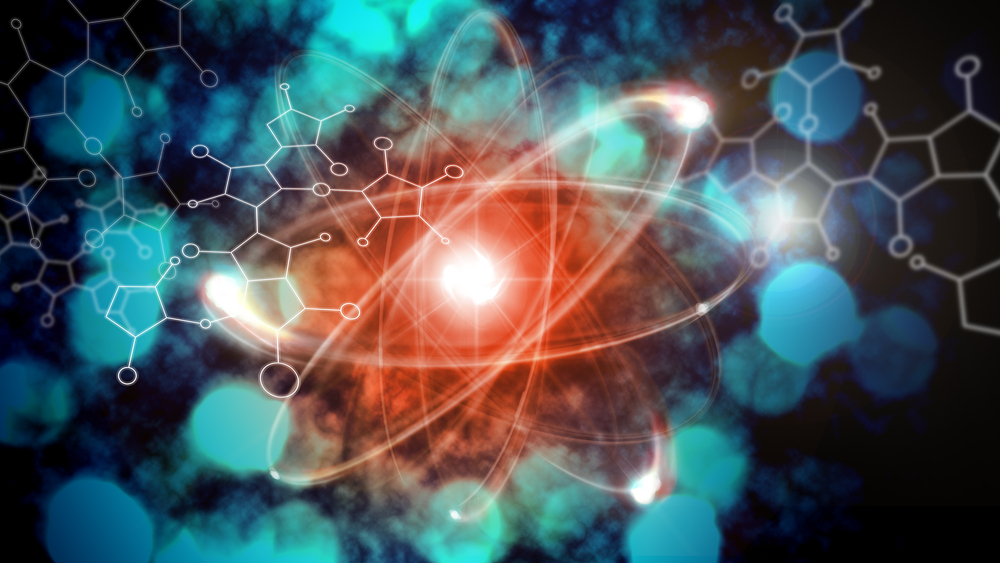
Deep inside a mountain in central Italy, scientists are laying a trap for dark matter. The bait? A big metal tank full of 3.5 tons (3,200 kilograms) of pure liquid xenon. This noble gas is one of the cleanest, most radiation-proof substances on Earth, making it an ideal target for capturing some of the rarest particle interactions in the universe.
It all sounds vaguely sinister; said Christian Wittweg, a doctoral candidate at the University of Münster in Germany, who has worked with the so-called Xenon collaboration for half a decade, going to work every day feels like "paying a Bond villain a visit." So far, the mountain-dwelling researchers haven't captured any dark matter. But they recently succeeded in detecting one of the rarest particle interactions in the universe. [11 Biggest Unanswered Questions About Dark Matter]
According to a new study published today (April 24) in the journal Nature, the team of more than 100 researchers measured, for the first time ever, the decay of a xenon-124 atom into a tellurium 124 atom through an extremely rare process called two-neutrino double electron capture. This type of radioactive decay occurs when an atom's nucleus absorbs two electrons from its outer electron shell simultaneously, thereby releasing a double dose of the ghostly particles called neutrinos.
By measuring this unique decay in a lab for the first time, the researchers were able to prove precisely how rare the reaction is and how long it takes xenon-124 to decay. The half-life of xenon-124 — that is, the average time required for a group of xenon-124 atoms to diminish by half — is about 18 sextillion years (1.8 x 10^22 years), roughly 1 trillion times the current age of the universe.
This marks the single longest half-life ever directly measured in a lab, Wittweg added. Only one nuclear-decay process in the universe has a longer half-life: the decay of tellurium-128, which has a half-life more than 100 times longer than that of xenon-124. But this vanishingly rare event has only been calculated on paper.
A precious decay
As with the more common forms of radioactive decay, two-neutrino double electron capture occurs when an atom loses energy as the ratio of protons and neutrons in the atomic nucleus changes. However, the process is much pickier than more-common decay modes and depends on a series of "giant coincidences," Wittweg said. Having literal tons of xenon atoms to work with made the odds of these coincidences lining up much more likely.
Here's how it works: All xenon-124 atoms are surrounded by 54 electrons, spinning in hazy shells around the nucleus. Two-neutrino double-electron capture occurs when two of those electrons, in shells close to the nucleus, simultaneously migrate into the nucleus, crashing into one proton apiece and converting those protons into neutrons. As a byproduct of this conversion, the nucleus spits out two neutrinos, elusive subatomic particles with no charge and virtually no mass that almost never interact with anything.
Sign up for the Live Science daily newsletter now
Get the world’s most fascinating discoveries delivered straight to your inbox.
Those neutrinos fly off into space, and scientists cannot measure them unless they use extremely sensitive equipment. To prove that a two-neutrino double electron capture event has occurred, the Xenon researchers instead looked to the empty spaces left behind in the decaying atom.
"After the electrons are captured by the nucleus, there are two vacancies left in the atomic shell," Wittweg said. "Those vacancies are filled up from higher shells, which creates a cascade of electrons and X-rays."
Those X-rays deposit energy in the detector, which the researchers can clearly see in their experimental data. After one year of observations, the team detected close to 100 instances of xenon-124 atoms decaying this way, providing the first direct evidence of the process.
This new detection of the second-rarest decay process in the universe doesn't put the Xenon team any closer to finding dark matter, but it does prove the versatility of the detector. The next step in the team's experiments involves building an even larger xenon tank — this one capable of holding more than 8.8 tons (8,000 kg) of liquid — to provide even more opportunities to detect rare interactions, Wittweg said.
- Elementary, My Dear: 8 Little-Known Elements
- What's That? Your Physics Questions Answered
- 18 Times Quantum Particles Blew Our Minds
Originally published on Live Science.
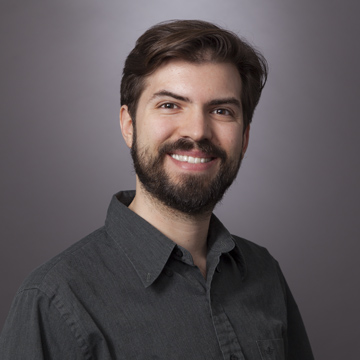
Brandon is the space/physics editor at Live Science. His writing has appeared in The Washington Post, Reader's Digest, CBS.com, the Richard Dawkins Foundation website and other outlets. He holds a bachelor's degree in creative writing from the University of Arizona, with minors in journalism and media arts. He enjoys writing most about space, geoscience and the mysteries of the universe.