What are antibodies?
Our body has a specialized search-and-destroy army. Antibodies are key players in that fight.
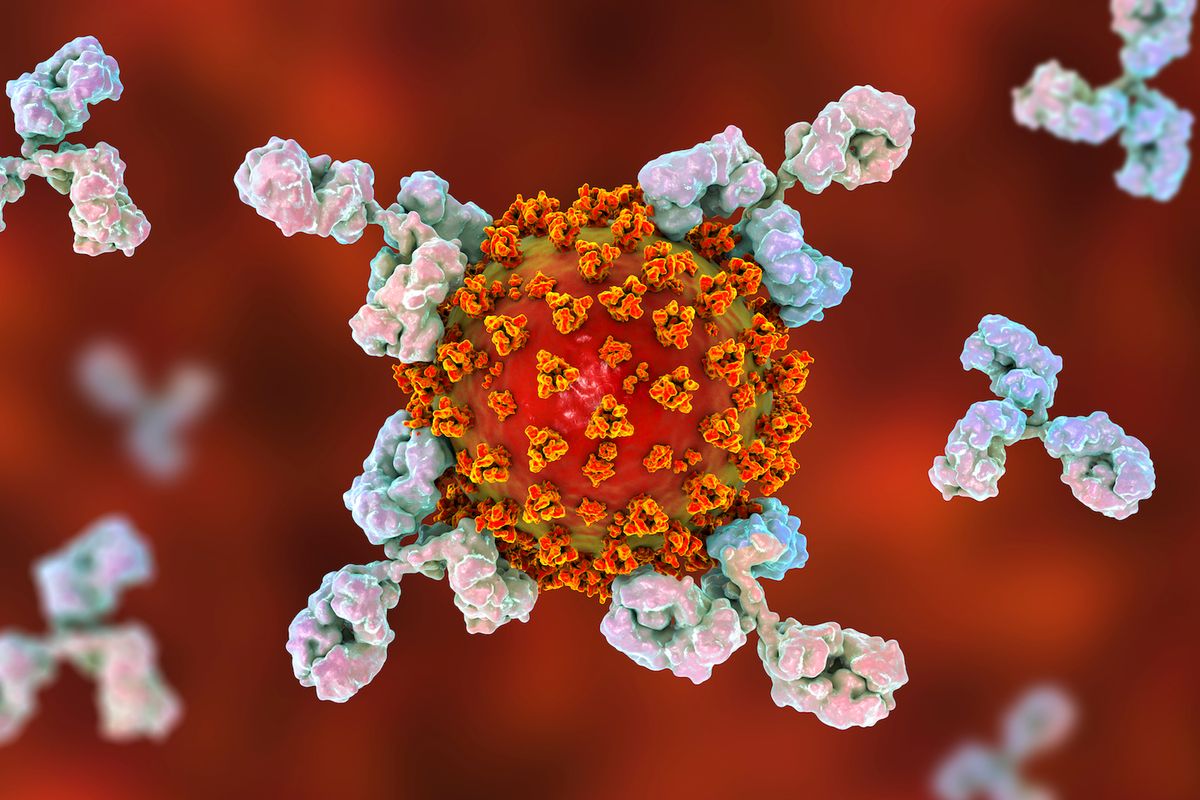
Antibodies are specialized, Y-shaped proteins that bind like a lock-and-key to the body's foreign invaders — whether they are viruses, bacteria, fungi or parasites. They are the "search" battalion of the immune system's search-and-destroy system, tasked with finding an enemy and marking it for destruction.
"They're released from the cell and they go out and hunt," said Dr. Warner Greene, the director of the Center for HIV Cure Research at the Gladstone Institutes in San Francisco.
When antibodies find their target, they bind to it, which then triggers a cascade of actions that vanquish the invader. Antibodies are part of the so-called "adaptive" immune system, the arm of the immune system that learns to recognize and eliminate specific pathogens, Greene said.
Related: Diagram of the human immune system (infographic)
What do antibodies look like?
The two arms at the top of the antibody's Y shape bind to what's known as the antigen. The antigen can be a molecule, or a molecular fragment — often some part of a virus or bacteria. (For instance, the new coronavirus SARS-CoV-2 has unique "spikes" on its outer coat, and some antibodies bind to and recognize these spike proteins.)
The bottom of the Y, or the stalk, binds to several other immune-system compounds that can help kill the antigen or mobilize the immune system in other ways. One set of these, for instance, triggers the complement cascade, Greene told Live Science.
"Complement is actually the executioner," that punches holes in the target cell, such as the membrane of a virus, Greene said.
Antibodies, which are also called immunoglobulins (Ig), all have the same basic Y-shape, but there are five variations on this theme — called IgG, IgM, IgA, IgD and IgE, said Jason Cyster, a professor of microbiology and immunology at the University of California, San Francisco.
Each variation looks slightly different and plays slightly different roles in the immune system. For instance, immunoglobulin G, or IgG, is just one Y, whereas IgM looks a bit like the 10-armed Hindu goddess Durga, with five Ys stacked together, and each prong can bind one antigen.
IgG and IgM are the antibodies that circulate in the bloodstream and go into solid organs, Cyster said. IgA is "squirted out of the body," in mucus or secretions, Cyster told Live Science. IgE is the antibody that typically triggers allergic responses, such as to pollen or peanuts, according to the American Academy of Allergy, Asthma & Immunology. IgD has historically been enigmatic, but one of its roles is to help activate the cells that make antibodies.
Related: Hay fever & seasonal allergies: Symptoms, causes & treatment
Where do antibodies form?
To understand antibodies, you first need to know about B-cells, which are a type of white blood cell that forms in the bone marrow. There are about a trillion B-cells in the body, and each one has a unique IgM antibody that sits on the B-cell surface and each binds, to one antigen, said Simon Goodman, the Science and Technology Program Manager for The Antibody Society, a nonprofit organization that represents those involved in antibody research and development.
This staggering level of variation allows the body to recognize almost any substance that could enter. Here’s how it achieves that diversity: In each B-cell, the genes that code for the antibody's binding site are shuffled like playing cards in a deck.
"The amount of rearrangement that can occur is enormous," Cyster told Live Science.
Related: 11 surprising facts about the immune system
These B-cells then patrol the body, often lingering longer in areas like the lymph nodes or the tonsils, Cyster said. Most of the time, these B-cells don't bind anything. But if, by a one- in-a-million chance, a B-cell does bind some foreign substance, "that triggers the B-cell to say 'Hey we need to get activated,'" Cyster said.
The B-cell grows in size and starts to divide in what's called "clonal expansion," Cyster said.
"It's an identical copy of the parent, just like the mother," Cyster said. After a week or so, there may be hundreds of thousands to a million of these copies.
Eventually, these clonally expanded B-cells differentiate into plasma cells, which are antibody factories.
"They secrete 10,000 antibodies per cell per second. They can do that for weeks or years if you're lucky," Cyster said.
But not all B-cells divide the same amount.
"If you consider the B-cell to be a lock, and you consider all of these different things to be floating around to be different keys, then some of the keys will fit better, some will fit worse, and some won't fit at all," Goodman told Live Science. "And depending on how well the key fits into the lock on the surface of a particular B-cell, that cell will be triggered to divide more." Then, the more prolific B-cells produce more plasma cells and churn out more of a specific type of antibody.
The body doesn't just produce one type of antibody either; it produces a messy, chaotic zoo of them. Each locks onto different parts of an invader.
And antibodies don't all do the same thing once they've bound to a target. Some will nip infection in the bud by directly neutralizing a threat, preventing a pathogen from entering a cell. Others tag invaders, so that the immune system's killer cells (which aren’t antibodies) can remove it, Greene said. Still others may wrap viruses or bacteria in a gooey coating. And other antibodies might tell Pac-Man-like immune cells called macrophages to come gobble up the invader. (That strategy can sometimes backfire with viruses, which may co-opt this response to invade new cells, Cyster added.)
The first type of antibody to form after you are exposed to a virus is IgM, which emerges within 7 to 10 days after exposure, Greene said. IgM can bind to an invader, but each "Y" in this 10-armed protein does so fairly weakly. But, just as five weak people working together can tackle a large, strong adversary, IgM's five Y's (10 arms) working together can bind tightly to an antigen, he added.
At about 10 to 14 days, the body begins making IgG, which is the immune system's "major workhorse," Greene said. IgG can cross the placenta in a pregnant woman, giving a newborn passive protection against disease until their own immune system can ramp up, Greene added.
Normally, the immune system is stunningly good at recognizing the enemy and ignoring, or tolerating, our own cells. Sometimes, however, this process goes awry. That’s when T-cells (another type of white blood cells) come in. The body uses these T-cells to cross-check targets — only if both a B-cell and a T-cell recognize something as a foreign invader will an immune response be triggered, Goodman said. The body is supposed to remove B-cells that make so-called auto-antibodies, which react to the body's own cells. But when that doesn't happen, the body may mark its own cells for destruction and then relentlessly eliminate them. Autoimmune diseases such as lupus, rheumatoid arthritis, or type 1 diabetes can result, Goodman said. There are more than 100 autoimmune disorders, according to the American Autoimmune Related Diseases Association.
What are monoclonal antibodies?
Antibodies have become the basis for some of the most useful medicines, as well as some of the most powerful lab techniques in biology, Goodman said. One of these clinical and therapeutic superstars is what's known as a monoclonal antibody.
To create a monoclonal antibody, researchers vaccinate an animal (or possibly a human) to stimulate the production of antibodies against a particular substance. The body will gradually make antibodies that are more and more effective against that antigen. These antibody-producing cells are then filtered out of white blood cells and put into a dish to see which cells bind the antigen best, Goodman said. The cell that binds the best is then isolated — it is an antibody-producing factory, specifically honed to churn out one super-selective antibody.
From there, that cell is fused to a blood cancer cell, producing something called a hybridoma. This hybridoma, or monoclone, is an inexhaustible generator of exactly the same antibody, over and over and over. (Researchers bind the monoclonal cell to a cancer cell because cancer just continues to reproduce.)
"It just produces and produces and produces, and it will never stop, and it's a cancer, so it's essentially immortal," Goodman said. What it produces is a monoclonal antibody.
Related: Ebola survivor's blood could lead to future vaccine
Such cell lines have an incredibly diverse range of uses. There are millions of commercial monoclonal antibodies, which are used in labs to tag the tiniest, most specific cellular targets for study, Goodman said.
"They're incredible, they're amazingly precise tools," Goodman said.
Monoclonal antibodies also form the basis for many blockbuster drugs. For instance, the drug adalimumab (brand name Humira), is a monoclonal antibody that treats rheumatoid arthritis by inhibiting an inflammatory protein known as a cytokine. Another, called bevacizumab (Avastin), targets a molecule that fuels blood vessel growth; by blocking this molecule, bevacizumab can slow the growth of lung, colon, kidney and some brain cancers.
And in the SARS-CoV-2 pandemic, doctors around the world are racing to create monoclonal antibodies that will hopefully neutralize the new coronavirus, Greene said. These antibodies are filtered from the plasma of people who have recovered from COVID-19 (also called convalescent serum). The hope is that by isolating the most effective antibodies, and then producing them en-masse, doctors can create a treatment that provides a temporary, "passive" immunity until the body can catch up and mount an effective, more long-lasting response on its own, Greene said.
Related: 1 in 5 people tested in New York have antibodies to the coronavirus
By contrast, polyclonal antibodies are derived from multiple B-cells. Polyclonal antibodies are a library of antibodies that all bind to slightly different parts of the antigen, or target. Polyclonal antibodies are typically produced by injecting an animal with the antigen, stimulating an immune response, and then extracting the animals' plasma to produce antibodies en masse, according to a 2005 study in the Institute for Laboratory Animal Research (ILAR) journal.
Unlike monoclonal antibodies, which can take up to 6 months to produce, polyclonal antibodies can be made in 4 to 8 weeks, and require less technical expertise. In addition, for certain types of tests where you are trying to detect the antigen, polyclonal antibodies might have a better chance of binding to the target antigen, making them potentially more sensitive. The downside of polyclonal antibodies is that, because each individual animal might produce a different array of antibodies, making polyclonal antibodies that are consistent from batch to batch can be more challenging, and it isn't as easy to have a large supply, according to a 2005 study in the journal Biotechniques.
How do antibody tests work?
Antibody tests detect whether the body has produced detectable quantities of antibodies to a certain molecule, and can therefore reveal whether someone has been infected by a specific virus or bacteria in the past. Usually, these tests are detecting IgM or IgG, Live Science previously reported.
For instance, SARS-CoV-2 antibody tests typically detect either part or all of the coronavirus' spike protein and can reveal whether someone has had COVID-19 in the past. Because the body takes time to ramp up its production of antibodies, people usually only test positive about two weeks after they were first exposed to the pathogen, Live Science previously reported.
Related: Can antibody tests tell if you're immune to COVID-19?
There are two common types of antibody tests — lateral flow assays and enzyme-linked immunosorbent assay (ELISA) tests. Both involve fixing an antigen to a surface and then detecting whether an antibody binds to that antigen. Usually, a chemical reaction, such as fluorescence or a color-change, is triggered when the antibody binds to the antigen. Lateral flow assays are similar to pee-on-a-stick pregnancy tests; rather than pee, for antibody tests, blood or serum is washed over the flat surface, which is usually paper. ELISA tests work on a similar principle, only the tests are conducted in microplates and require a lab technician, and the results may not read out instantly, Charlotte Sværke Jørgensen, who studies Virus and Microbiological Special Diagnosis Serology at the Statens Serum Institut in Copenhagen, previously told Live Science in an email.
A good antibody test is one that produces few false positives and few false negatives, Live Science previously reported. To ensure that happens, scientists need to "calibrate" their test, for instance, by making sure that samples known to not have the antigen do not falsely produce a positive test. For instance, with SARs-CoV-2, that would mean testing blood samples from before the pandemic started and making sure no samples come up positive. They also need to take samples that definitely have the antibody in them, and make sure the antibody test does a good job of detecting those positives.
Additional resources:
- Watch a video about how antibodies work, produced by the Vaccine Makers Project.
- Read more about antibody tests from the Centers for Disease Control and Prevention.
- Read what the director of the National Institutes of Health, Dr. Francis Collins, has to say about SARS-CoV-2 antibodies, as published on the NIH director's blog on June 30, 2020.
Sign up for the Live Science daily newsletter now
Get the world’s most fascinating discoveries delivered straight to your inbox.
Tia is the managing editor and was previously a senior writer for Live Science. Her work has appeared in Scientific American, Wired.com and other outlets. She holds a master's degree in bioengineering from the University of Washington, a graduate certificate in science writing from UC Santa Cruz and a bachelor's degree in mechanical engineering from the University of Texas at Austin. Tia was part of a team at the Milwaukee Journal Sentinel that published the Empty Cradles series on preterm births, which won multiple awards, including the 2012 Casey Medal for Meritorious Journalism.