
World's largest atom smasher could seed microscopic black holes
And reveal extra dimensions.
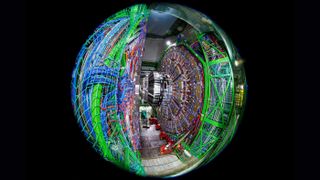
The cosmos may be studded with black holes so tiny they could slip in between atoms, a wild new theory suggests.
And we could be making these teensy singularities all the time at the world's largest atom smasher, a new study shows. If we could make these objects, they could be a window into the mysterious nature of gravity.
Related: Photos: The world's largest atom smasher
One size too small
We have four fundamental forces of nature (at least, that we know about so far): electromagnetism, strong force, weak force and gravity. All four forces operate at different ranges, have different carriers and interact in different ways. They also have very different strengths.
And no matter how you slice it, gravity always comes out the weakest. Gravity is such a feeble force that if it were a billion billion times stronger than it currently is, it would still be the weakest force by a factor of about 10 million.
Go ahead and lift whatever device you're reading on above your head. Congratulations. The muscles in your arm temporarily overcame the gravitational pull of the entire planet Earth.
Gravity is so laughably weak that physicists have started to wonder why. After all, something that strange, that odd, that out-of-place is practically begging for an explanation.
Sign up for the Live Science daily newsletter now
Get the world’s most fascinating discoveries delivered straight to your inbox.
Related to this gravity-is-so-weirdly-weak problem (known as the "hierarchy problem" in physics circles) is another problem with how the fundamental forces are tied together.
At high energies, the electromagnetic and weak nuclear forces merge together into a single, unified force (called, of course, the "electroweak" force). We have evidence that at even higher energies, the strong nuclear force also joins the unification party. And it's hypothesized that at exceedingly high energies, gravity too merges with the other forces. But the required energy is so high that we couldn't hope to reach it, even with a particle accelerator the size of our galaxy.
Why is gravity so weak, and why does it take so much energy to potentially merge it with the other forces?
A flood of darkness
The short version is that we don't know. One hypothesis is that there is more to the universe than meets the eye. Specifically, there are more spatial dimensions than the three we know of. In this view, there's the usual up-down, left-right, and back-forth directions, plus … some others. The exact number depends on the theory.
In this potential explanation for the weakness of gravity, the extra dimensions are so large that our universe is embedded in a much vaster, higher-dimensional bulk made of the extra dimensions.
In this story, gravity is actually very strong, but unlike the other forces (which are constrained to our 3D universe), gravity gets to roam freely among all the dimensions. This dilutes the force, making it seem wea.
In these models, since gravity is actually so strong, it would be possible to merge gravity at much lower energy levels. In other words, we may not need a galaxy-sized particle accelerator to see gravity join the unification party. We may even only need something far smaller. Like, say, the Large Hadron Collider, a 16.5-mile-long (27 kilometers) ring on the border of France and Switzerland, where protons are sent smashing into each other at near light-speed.
The rules of the game
How would the LHC spot those hidden extra dimensions? One way would be through the production of microscopic black holes. It normally requires an insane amount of density and pressure to form a black hole –- compressing matter so that it collapses to a point of infinite density is no easy trick. And if gravity truly is as weak as it seems, then we don't have nearly enough energy inside the LHC to make it happen.
Related: The biggest black hole findings
But if the force of gravity is actually a lot stronger, we could easily overwhelm the other forces of nature and produce black holes. If gravity is strong enough, we could already be manufacturing tiny black holes in the LHC right now.
These tiny black holes are not a cause for alarm — they would evaporate in less than 10^minus 27 seconds, transmuting into a shower of particles well before they did anything interesting like swallowing Earth. But to date we haven't seen any of particle sprays suggestive of microscopic black holes or extra dimensions.
But in a paper accepted to be published in the journal Physical Review D and posted to the preprint database arXiv, a team of theoretical physicists have pointed out that we may be missing something. Previous calculations of how often the LHC would create microscopic black holes made some simple assumptions about how the black holes would form and how they would interact with the universe around them.
This group's more precise calculations reveal that, assuming all those extra dimensions exist and gravity is secretly superstrong, the LHC can produce far fewer microscopic black holes than we previously realized. Depending on the number of hypothetical extra dimensions, the number of these teensy black holes could be as low as one-tenth of the previous estimates.
This means that all hope is not lost in the search of extra dimensions and gravity's potential hidden strength. We may just have to keep running the LHC longer before we can rule these models out.
And if a microscopic black hole does make an appearance in our data, it would mean that what we think of as the universe is just a small bubble embedded in a much larger framework — and we would have to completely rewrite our understanding of gravity.
Originally published on Live Science.
Paul M. Sutter is a research professor in astrophysics at SUNY Stony Brook University and the Flatiron Institute in New York City. He regularly appears on TV and podcasts, including "Ask a Spaceman." He is the author of two books, "Your Place in the Universe" and "How to Die in Space," and is a regular contributor to Space.com, Live Science, and more. Paul received his PhD in Physics from the University of Illinois at Urbana-Champaign in 2011, and spent three years at the Paris Institute of Astrophysics, followed by a research fellowship in Trieste, Italy.