'A dream come true': Nuclear clock breakthrough could revolutionize study of the universe's fundamental forces
By nudging a thorium-229 nucleus into a higher energy state, physicists have made it possible to develop a nuclear clock that could probe the most fundamental forces in physics. However, there is still a long way to go.
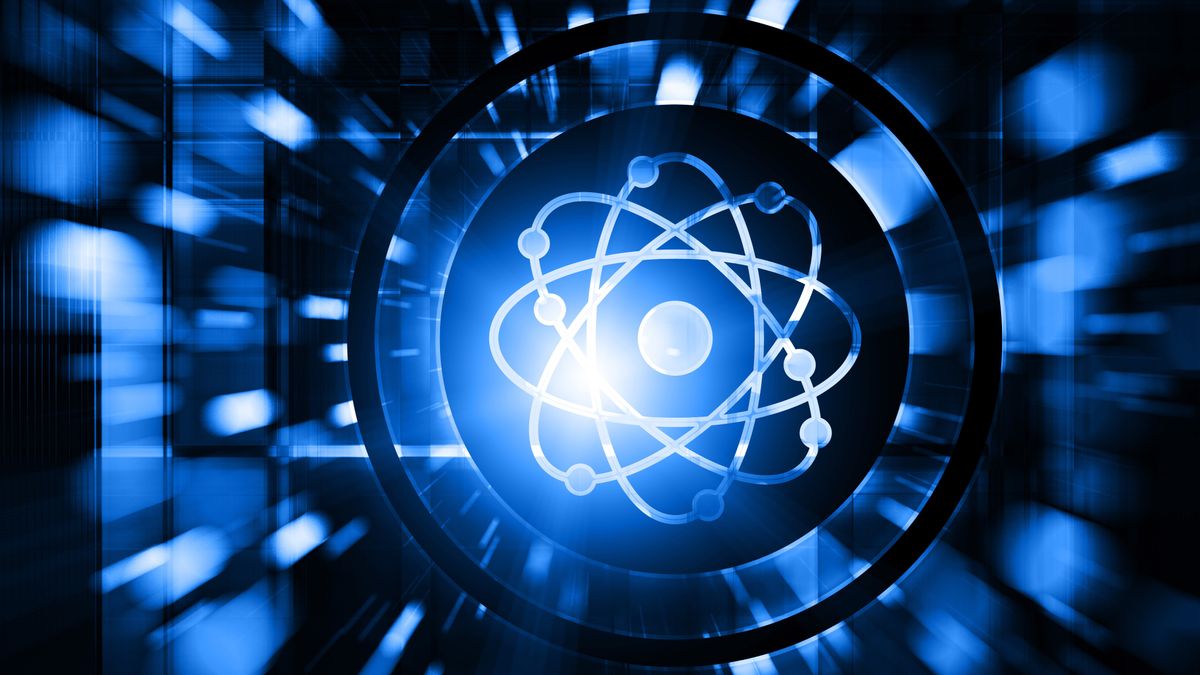
Scientists have made a major breakthrough that takes us a step closer to developing a nuclear clock — a device that keeps time based on the inner workings of atoms.
For the first time, physicists have used laser light to bump the nucleus of a thorium atom up to a higher energy level. The discovery paves the way for the development of a new clock whose ticks are not only more precise but can probe the most fundamental forces in the universe.
The researchers published their findings April 29 in the journal Physical Review Letters.
"Seeing the first signal was a dream come true," lead researcher Thorsten Schumm, a professor of quantum metrology at the Vienna University of Technology, told Live Science. "[It's] the reward for many years of preparation, while also doubting whether this would actually ever work."
About time
Currently, our most accurate clocks are atomic and keep time by firing lasers at electrons — matching the laser's frequency with the precise jumps across energy levels it causes electrons orbiting atoms to make. This method gives scientists an ultraprecise measurement of the laser's frequency, from which they can extract the "tick" of the atomic clock.
However, atomic clocks are far from perfect. The electrons they rely on to keep time sit outside atoms. They are therefore vulnerable to interference from stray magnetic fields or other environmental effects that can subtly alter their energy levels, the frequency of laser light they subsequently respond to, and therefore the time they keep.
Related: Otherworldly 'time crystal' made inside Google quantum computer could change physics forever
Sign up for the Live Science daily newsletter now
Get the world’s most fascinating discoveries delivered straight to your inbox.
A nuclear clock, on the other hand, would use the energy transitions of nuclei inside the heart of an atom, so they are shielded from outside interference. But many of the gaps between nuclei energy levels are thousands of times greater than those for electrons — meaning they are too large to be crossed with the energy of a laser.
But in the 1970s, scientists found that one isotope, or version, of the element thorium (thorium-229) seemed to have an energy level that could be spanned by laser light.
But finding this precise energy gap has been no simple task. Initially, researchers excited thorium-229 to an energy level far above the two that physicists were actually interested in. They then measured the subtle differences in the energy of light emitted when it fell back down to the higher one compared to the one just below it.
The researchers have compared this process to finding the height of a kerb by dropping balls from a skyscraper — the subtle differences in bounce heights when the ball hits the street to when it hits the sidewalk can help them zero in on the small distance between them.
Over the past 50 years, research narrowed the energy required to cause this energy level jump to the tiny fractions of an electron volt — but this precision was still not enough.
"Theory tells us that it was somewhere in the energy range between 0eV and 10 eV, but we need to hit the right frequency with 7 to 8 digit precision to cause an effect," Schumm said. "Scanning the entire search range would take millenia, so we had to narrow down the search range over many years of preparatory experiments."
To finally hone in on the precise value, Schumm and his team trapped around 10 to the power of 17 thorium-229 nuclei (or a million times more nuclei than there are stars in our galaxy) inside crystals of calcium fluoride, which greatly increased the likelihood of finding the desired transition. After many attempts, the researchers directly observed a thorium atom leaping between the energy levels: an energy change of 8.35574 electron volts.
The researchers note that it will take many more years to develop nuclear clocks to the same accuracy of their atomic counterparts. But with this transition finally spotted, the window has finally been opened, and it could enable physicists to probe more deeply into the elusive nature of dark energy, dark matter and the fundamental forces of our universe.
"The nuclear clock will provide an extremely precise measurement of the energy difference between two bound states of the nucleus," Schumm said. "These two binding energies are the result of three out of the four fundamental forces in physics: electromagnetism, the strong nuclear force, and the weak nuclear force. This is in contrast to all atomic clocks, which rely on electromagnetism alone. If one of these three fundamental forces changes as a function of time or location in space, the nuclear clock should see this."
Ben Turner is a U.K. based staff writer at Live Science. He covers physics and astronomy, among other topics like tech and climate change. He graduated from University College London with a degree in particle physics before training as a journalist. When he's not writing, Ben enjoys reading literature, playing the guitar and embarrassing himself with chess.