Quantum physicists discover 'negative time' in strange experiment
Physicists showed that photons can seem to exit a material before entering it, revealing observational evidence of negative time
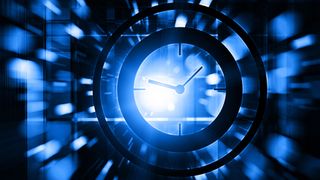
Quantum physicists are familiar with wonky, seemingly nonsensical phenomena: atoms and molecules sometimes act as particles, sometimes as waves; particles can be connected to one another by a "spooky action at a distance," even over great distances; and quantum objects can detach themselves from their properties like the Cheshire Cat from Alice's Adventures in Wonderland detaches itself from its grin. Now researchers led by Daniela Angulo of the University of Toronto have revealed another oddball quantum outcome: photons, wave-particles of light, can spend a negative amount of time zipping through a cloud of chilled atoms. In other words, photons can seem to exit a material before entering it.
"It took a positive amount of time, but our experiment observing that photons can make atoms seem to spend a *negative* amount of time in the excited state is up!" wrote Aephraim Steinberg, a physicist at the University of Toronto, in a post on X (formerly Twitter) about the new study, which was uploaded to the preprint server arXiv.org on September 5 and has not yet been peer-reviewed.
The idea for this work emerged in 2017. At the time, Steinberg and a lab colleague, then doctoral student Josiah Sinclair, were interested in the interaction of light and matter, specifically a phenomenon called atomic excitation: when photons pass through a medium and get absorbed, electrons swirling around atoms in that medium jump to higher energy levels. When these excited electrons lapse to their original state, they release that absorbed energy as reemitted photons, introducing a time delay in the light's observed transit time through the medium.
Sinclair's team wanted to measure that time delay (which is sometimes technically called a "group delay") and learn whether it depends on the fate of that photon: Was it scattered and absorbed inside the atomic cloud, or was it transmitted with no interaction whatsoever? "At the time, we weren't sure what the answer was, and we felt like such a basic question about something so fundamental should be easy to answer," Sinclair says. "But the more people we talked to, the more we realized that while everyone had their own intuition or guess, there was no expert consensus on what the right answer would be." Because the nature of these delays can be so strange and counterintuitive, some researchers had written the phenomenon off as effectively meaningless for describing any physical property associated with light.
After three years of planning, his team developed an apparatus to test this question in the lab. Their experiments involved shooting photons through a cloud of ultracold rubidium atoms and measuring the resulting degree of atomic excitation. Two surprises emerged from the experiment: Sometimes photons would pass through unscathed, yet the rubidium atoms would still become excited — and for just as long as if they had absorbed those photons. Stranger still, when photons were absorbed, they would seem to be reemitted almost instantly, well before the rubidium atoms returned to their ground state — as if the photons, on average, were leaving the atoms quicker than expected.
The team then collaborated with Howard Wiseman, a theoretical and quantum physicist at Griffith University in Australia, to devise an explanation. The theoretical framework that emerged showed that the time these transmitted photons spent as an atomic excitation matched perfectly with the expected group delay acquired by the light — even for cases where it seemed as though the photons were reemitted before the atomic excitation had ebbed.
Related: Time might be a mirage created by quantum physics, study suggests
Sign up for the Live Science daily newsletter now
Get the world’s most fascinating discoveries delivered straight to your inbox.
To understand the nonsensical finding, you can think of photons as the fuzzy quantum objects they are, in which any given photon's absorption and reemission through an atomic excitation is not guaranteed to occur over a certain fixed amount of time; rather, it takes place across a smeared-out, probabilistic range of temporal values. As demonstrated by the team's experiments, these values can encompass instances when an individual photon's transit time is instantaneous — or, bizarrely, when it concludes before the atomic excitation has ceased, which gives a negative value.
"I can promise you that we were completely surprised by this prediction," Sinclair says, referring to the matchup between the group delay and the time that the transmitted photons spent as atomic excitations. "And as soon as we were confident we hadn't made a mistake, Steinberg and the rest of the team — I had moved on to do a postdoc at [the Massachusetts Institute of Technology] by this point — began planning to do a follow-up experiment to test this crazy prediction of negative dwell time and see if the theory would hold up."
That follow-up experiment, the one led by Angulo that Steinberg touted on X, can be understood by considering the two ways a photon can be transmitted. In one, the photon wears blinders of sorts and ignores the atom entirely, leaving without even a nod. In the other, it interacts with the atom, boosting it to a higher energy level, before getting reemitted.
"When you see a transmitted photon, you can't know which of these occurred," Steinberg says, adding that because photons are quantum particles in the quantum realm, the two outcomes can be in superposition—both things can happen at the same time. "The measuring device ends up in a superposition of measuring zero and measuring some small positive value." But correspondingly, Steinberg notes, that also means that sometimes "the measuring device ends up in a state that looks not like 'zero' plus 'something positive' but like 'zero' minus 'something positive,' resulting in what looks like the wrong sign, a negative value, for this excitation time."
The measurement results in Angulo and her colleagues' experiment suggest that the photons moved through the medium faster when they excited the atoms than when the atoms remained in their ground state. (The photons aren't communicating any information, so the outcome does not contradict the "nothing can travel faster than light" speed limit set by Einstein's special theory of relativity.)
"A negative time delay may seem paradoxical, but what it means is that if you built a 'quantum' clock to measure how much time atoms are spending in the excited state, the clock hand would, under certain circumstances, move backward rather than forward," Sinclair says. In other words, the time in which the photons were absorbed by atoms is negative.
Even though the phenomenon is astonishing, it has no impact on our understanding of time itself — but it does illustrate once again that the quantum world still has surprises in store.
"[Angulo] and the rest of the team have accomplished something really impressive and produced a beautiful set of measurements. Their results raise interesting questions about the history of photons traveling through absorptive media and necessitate a reinterpretation of the physical meaning of the group delay in optics," Sinclair says.
A version of this article originally appeared in Spektrum der Wissenschaft and was reproduced with permission.
This article was first published at Scientific American. © ScientificAmerican.com. All rights reserved. Follow on TikTok and Instagram, X and Facebook.
Manon Bischoff studied physics at the TU Darmstadt (with a semester abroad in Buenos Aires) and then worked as a research assistant at the JGU Mainz. From there, she initially ended up as an intern at Spektrum der Wissenschaft in 2017 and, after a traineeship, became an editor for the areas of mathematics, computer science and physics.