Room-temperature superconductors: The facts behind the 'holy grail' of physics
Claims of a room-temperature superconductor went viral last week. Here's everything we know.
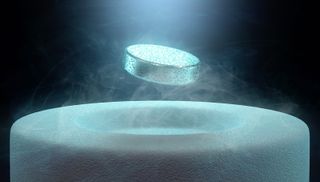
Since their first discovery in 1911, superconductors — materials which perfectly conduct electricity — have long beguiled and tantalized physicists.
Superconductors are used in particle accelerators, nuclear fusion devices, MRI machines, and even maglev trains. Yet broader and more common applications are hampered by a forbidding temperature limit — so far, no superconductor has been proven to work at ambient pressures and temperatures.
On July 22, scientists in South Korea published research claiming to have solved this problem. They say that their material, called LK-99, has an electrical resistivity, or resistance to the flow of electrical current, that drops to near zero at 30 degrees Celsius (86 degrees Fahrenheit). Their claims have sparked a global race to recreate the material and test its properties. As of August 4, no one has yet been able to replicate the results.
Here’s everything you need to know about superconductors.
What is superconductivity?
All materials possess a property known as resistivity — try to send an electrical current through them, and some of the energy in the current inevitably gets lost. This is because the current-carrying electrons collide with ions jiggling inside the material, creating opposition to their flow.
But cool a material down so that the ions inside it have less energy to vibrate, and the collision rates drop, sharply lowering the resistivity. Most materials would need to get to the impossible-to-reach state of absolute zero to have zero resistivity, yet some rare materials can attain zero resistivity above absolute zero temperatures — we call these materials superconductors.
The first superconductor was discovered in 1911 after the Dutch physicist Heike Kamerlingh Onnes noticed that a supercooled mercury wire (its temperature lowered to roughly minus 452 F (minus 269 C) no longer resisted the flow of electricity; an observation which won him the Nobel Prize in Physics. His observations were soon made using other elements such as lead, niobium, and tin.
Sign up for the Live Science daily newsletter now
Get the world’s most fascinating discoveries delivered straight to your inbox.
How do superconductors work?
Despite Onnes’s discovery, explaining why it happened would take decades.
The explanation finally emerged in 1957 under the name of the Nobel Prize winning “BCS theory”. Named after its discoverers John Bardeen, Leon Cooper and John Robert Schrieffer, BCS theory explained that superconductivity emerged from ripples caused by electrons as they moved through the material. At low enough temperatures, these ripples cause atomic nuclei inside atoms to become drawn to each other, in turn causing a slight offset in charge that attracts a second electron to the first. The force of this attraction causes something strange to happen: Instead of repelling each other through the force of electrostatic repulsion, the electrons instead get bound together into a “Cooper pair.”
Cooper pairs follow different quantum mechanical rules from those of lonesome electrons. Instead of stacking on top of each to form energy shells, they act like particles of light — an infinite number of which can occupy the same point in space at the same time. Create enough of these Cooper pairs throughout a material, and they become a superfluid, flowing without any loss of energy. Stir a superfluid once, and it will theoretically remain swirling until the end of the universe.
But this was far from the final surprise superconductivity had in store for physicists. In 1986, the late Alex Müller and Georg Bednorz, both of IBM, found that materials called cuprates — made up of layers of copper and oxygen sandwiched between other elements — could superconduct at temperatures as high as minus 211 F (minus 135 C).
Exactly why this happens is still not totally understood, but the dominant theory is one proposed by the American physicist Phillip Anderson, who suggested that electrons will choose to switch places with each other through a quantum mechanical process called superexchange.
Electrons constantly seek to switch places because, much like all particles and many things in nature, they seek to occupy the lowest possible energy state. As Heisenberg’s uncertainty principle states that only a particle’s position or momentum can be clearly known at one time, the electrons move to make their positions the most uncertain and their momentum the most clearly defined.
This constant switching, in turn, means that the electrons’ energies can be more sharply defined, enabling them to sink down into the lowest possible energy state. And the ideal configuration for this switching to take place? It just so happens to be a sea of evenly-spaced Cooper pairs.
Some recent experiments have suggested that Anderson was right — at least in the materials they studied — yet superexchange could theoretically be just one type of electron glue among many. Just as uncertain is how high a temperature some of these hypothetical electron glues could operate at, and what manufactured materials could produce these electron glues.
Superconductors have one telltale property: levitation. Because a flowing current generates a magnetic field, as materials transition to superconductive states the electrons inside flow without friction, generating a magnetic field that can repel an external magnet with an equal and opposite force. Place a superconductor above a magnet and it will be perfectly suspended in the air, a phenomenon called the Meissner effect.
Are room temperature superconductors possible?
Room temperature superconductors don't violate any known physics theories, but neither do any theories predict them.
The difficulty of creating them boils down to an engineering puzzle, with a forbidding array of atoms and chemical properties across many combinations of materials to test.
Among the materials scientists have tested is graphene, which can have its low-temperature superconductivity switched on or off depending on the twists and turns of its one-atom-thick sheets. Another promising candidate is the element scandium, a silvery metal which, this year, researchers reported can superconduct at warmer (but still very cold) temperatures.
Yet one infamous claim has left the field muddied in scandal. In a 2020 experiment, researchers said they observed a mixture of carbon, sulfur and hydrogen crushed to high pressures beneath two diamonds superconducting at a stunning 57 F (14 C). A follow-up experiment conducted this year upgraded the claim — for a scaled-up chunk of the material, the superconducting temperature was actually up to 70 F (21 degrees C). Following investigation by other scientists, however, the 2020 paper was retracted and the team behind the two experiments were accused of data manipulation and plagiarism.
Onto this crowded scene comes LK-99, a material whose resistivity, its researchers claim, drops to near zero at 86 F (30C). The material is made up of mixed powders containing lead, oxygen, sulfur and phosphorus which is doped with copper. It is also relatively easy to manufacture and test.
So far, 11 attempts to replicate the results have been announced by scientific institutions and seven have declared results. Of these seven, three have found properties similar to those claimed for LK-99, but not superconductivity. The remaining four observed neither magnetism nor superconductivity.
Ben Turner is a U.K. based staff writer at Live Science. He covers physics and astronomy, among other topics like tech and climate change. He graduated from University College London with a degree in particle physics before training as a journalist. When he's not writing, Ben enjoys reading literature, playing the guitar and embarrassing himself with chess.