'Strange metals' used in superconductors can entangle whole seas of electrons at once, and scientists finally understand how
Strange metals have baffled scientists since their discovery. Now, theorists have finally come up with an explanation.
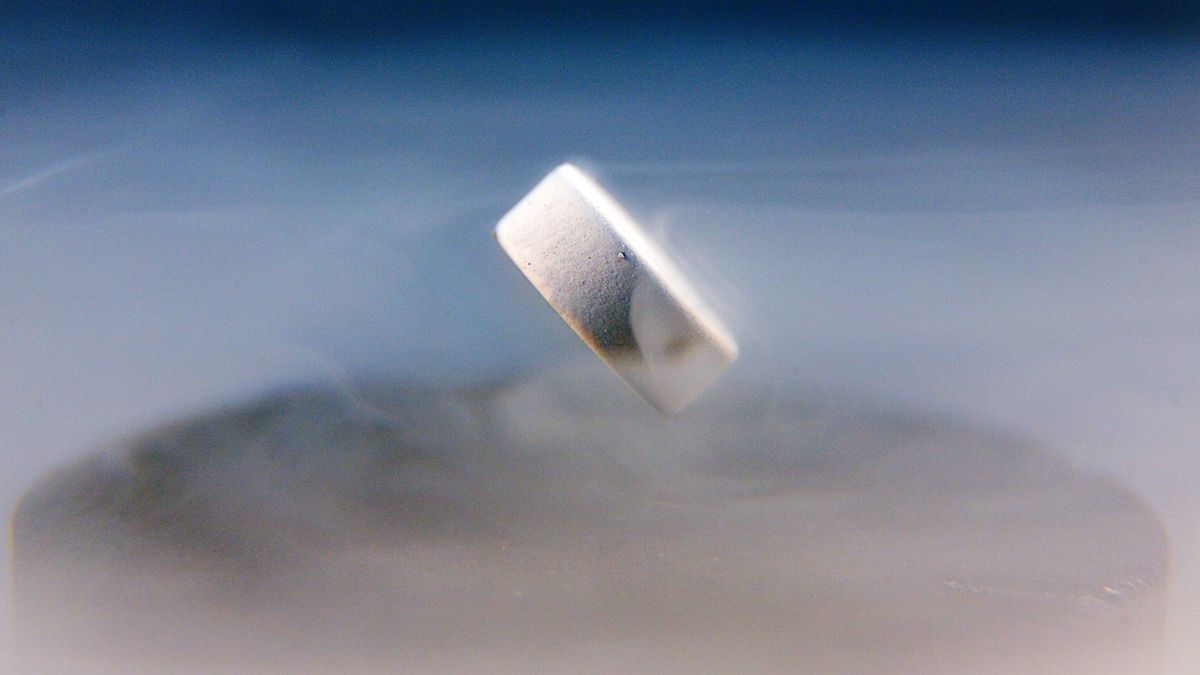
Physicists have come up with a universal theory to explain how a group of bizarre metals behave, and it could aid the search for a room-temperature superconductor.
"Strange metals" are a type of quantum material with some truly weird properties: Not only do they flout the rules of electrical resistance seen in regular metals, but some even have the ability to become superconductors at relatively high temperatures.
Yet strange metals have confounded physicists since their discovery 40 years ago, suggesting that a new fundamental theory is needed to understand how they work. Now, a new study, published Aug. 18 in the journal Science, claims to offer just that.
Related: Bizarre 'demon' particle found inside superconductor could help unlock a 'holy grail' of physics
"Traditional methods of solid state physics assume crystal lattices are homogenous, and do not consider how inhomogeneity can affect how electrons interact with each other," Aavishkar Patel, a physicist at the Flatiron Institute's Center for Computational Quantum Physics (CCQ), told Live Science. "But the randomness is key."
In regular metals, electrical resistivity (the measure of how difficult it is for an electrical current to flow through a material) increases with the square of temperature before flattening out when the metal gets very hot.
This makes intuitive sense — resistivity arises when charge-carrying electrons in a metal collide and scatter within the metal's vibrating atomic structure, so increasing the vibrations of the atoms will increase this scattering rate up to the point where the electrons become unable to carry a current.
Sign up for the Live Science daily newsletter now
Get the world’s most fascinating discoveries delivered straight to your inbox.
But in 1986, a class of copper-containing materials called cuprates broke all the rules. The resistance of cuprates increased linearly with temperature, and when some of them were cooled below a certain temperature threshold, they transformed into superconductors.
The superconductivity of cuprates is a tantalizing feature. Unlike the first superconductors discovered, which transition into having zero electrical resistivity near absolute zero (minus 459.67 Fahrenheit, or minus 273.15 Celsius) cuprates become superconductors at minus 211 F (minus 135 C).
Physicists hoped this discovery would lead to finding room temperature superconductors — opening the door to the near-lossless transmission of electricity. Yet the discoveries petered out, and recent claims of room temperature superconductors have ended in scandal and disappointment.
To better describe strange metals, the researchers in the new study devised a theory for how they work. Instead of the traditional view of metals — which says they consist of a sea of largely individual electrons in a uniform structure — the new theory proposes that strange metals are made of many electrons bound together by spooky rules of quantum entanglement, and these electrons swim through a random atomic patchwork.
"We have entanglement between many electrons all at the same time, not just two in a pair. The system of electrons is 'quantum critical' — in a sense that means it's as entangled as it can possibly get," Patel said.
As the atoms in strange metal lattices are dotted around randomly, the electrons inside entangle differently depending on their locations within the metal. This causes them to bump into each other frequently but at different speeds — setting up choke points and jams within the material.
The researchers say that their new theory predicts many features of strange metals, such as the linear relationship between temperature and resistivity; the frequency dependence of their conductivity when placed inside electromagnetic fields; their specific heat capacities; and their response to "shot noise" experiments that probe the entangled nature of the electrons inside them.
To disprove the theory, strange metal behavior would need to be observed in a strange metal with no randomness in its lattice structure. In the meantime, by blocking competing ways the electrons can interact, the theory could "leave the door open" for the emergence of room temperature superconductors, the researchers said.
Ben Turner is a U.K. based staff writer at Live Science. He covers physics and astronomy, among other topics like tech and climate change. He graduated from University College London with a degree in particle physics before training as a journalist. When he's not writing, Ben enjoys reading literature, playing the guitar and embarrassing himself with chess.