'Final parsec problem' that makes supermassive black holes impossible to explain could finally have a solution
A new study helps solve the "final parsec problem" that has made supermassive black hole formation impossible to explain, pointing to a strange form of dark matter as the key.
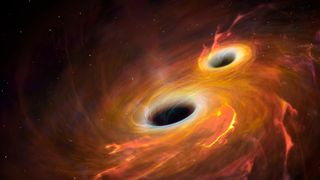
Scientists have been modeling how supermassive black holes form when two smaller black holes merge. But in their simulations, most pairs of massive black holes get stuck orbiting each other indefinitely. Now, scientists may have finally found a solution to this "final parsec problem" — and it may also help uncover the identity of one of the universe's most mysterious components.
Lurking at the heart of most ordinary galaxies is a supermassive black hole (SMBH), like the one imaged by the Event Horizon Telescope collaboration in the galaxy M87. That one is about 6.5 billion times the mass of the sun, but it wasn't always so big. Astronomers think SMBHs start out much smaller and grow into behemoths through repeated mergers with other black holes.
Evidence for those colliding giants came in 2023, when scientists with the International Pulsar Timing Array collaboration announced they had found a background "hum" of gravitational waves — ripples in the fabric of space-time released during mergers of extremely massive objects. Astronomers think this background is produced by distant pairs of massive black holes as they send space "ringing" with the gravitational echo of their faraway collisions.
Eternal cosmic dance
Researchers use sophisticated computer simulations to investigate the complex dance of these circling black holes. But until now, the models have run into a problem: When the black holes get down to a separation of about a parsec — about 3.26 light-years — they get stuck, circling each other eternally.
Related: Black hole singularities defy physics. New research could finally do away with them.
That's because, to collide and merge, the spiraling black holes must first lose energy and slow down. While approaching each other from many light-years apart, the black holes orbit through gas clouds and star clusters that slow their motion, causing them to spiral even closer.
But by the time they reach the last parsec, there isn't enough material left to drain their energy. Instead, the models predict that the duration of their final merger stretches to more than the current age of the universe. This has become known as the "final parsec problem."
Sign up for the Live Science daily newsletter now
Get the world’s most fascinating discoveries delivered straight to your inbox.
An extra ingredient
Scientists have come up with a few ideas to solve the problem. One answer could be that a spinning disk of matter that orbits the black holes, called an accretion disk, could speed their infall. Previous computer simulations show these reduce the time to a few billion years, but that's not enough to account for the observed background of gravitational waves or to explain how SMBHs can grow so large.
Now, a paper published in July in the journal Physical Review Letters suggests a new way black holes could lose this remaining energy: if dark matter is "self-interacting."
"The possibility that dark matter particles interact with each other is an assumption that we made, an extra ingredient that not all dark matter models contain," lead study author Gonzalo Alonso-Álvarez, a postdoctoral fellow at the University of Toronto, said in a statement. "Our argument is that only models with that ingredient can solve the final parsec problem."
Although dark matter is five times more abundant in the universe than ordinary matter, it is essentially invisible and little is known about its properties. Usually, scientists assume that it is collisionless, meaning it doesn't interact with ordinary matter or itself, in any way except through gravity. But because so little is known about it, astronomers sometimes move beyond this simple model.
Physicists have considered self-interacting dark matter (SIDM) before because it can help account for small-scale structures in galaxies that more traditional dark matter struggles with, and because it may help to explain the formation of unexpectedly large galaxies in the early universe.
The gravitational pull of SMBHs attracts dark matter into a dense concentration astrophysicists call a "spike." When the study authors used ordinary dark matter in their models, the spike did not absorb all of the energy from the black holes.
The "spikes are incapable of absorbing the frictional heat and are destroyed by the merger," the team explains in the paper. The energy from the orbiting black holes heats up the dark matter, eventually dispersing it into the wider galaxy, neutralizing the desired effect on the orbiting black holes.
However, when the team adjusted the properties of the dark matter in their models to make it self-interacting, they found that the spike absorbed the energy without being disrupted. The black holes continue to spiral inward and into the zone where they emit gravitational waves that pulsar timing experiments can detect. (Pulsars — rapidly rotating neutron stars — emit beams of radiation like cosmic lighthouses; by carefully measuring the arrival times of their flashes, scientists can detect tiny variations caused by the passage of gravitational waves).
In these models, the black holes merge in less than a billion years — a timescale short enough that countless mergers could produce the detected gravitational wave background.
SIDM softens the spectrum
While still theoretical, the new SIDM models may help solve another puzzle. When the black holes are far apart, they radiate very long gravitational waves, like widely separated crests of ocean waves. As the black holes spiral closer, the crests also get closer together. But measurements from pulsar timing hint that the height of the crests is smaller when they are closer together — an effect astronomers call a "softening" of the spectrum.
There is no such softening when they use ordinary dark matter, but when the team introduced SIDM instead, the dark matter spike not only absorbed energy but also softened the gravitational wave spectrum.
"A prediction of our proposal is that the spectrum of gravitational waves observed by pulsar timing arrays should be softened at low frequencies," study co-author James Cline, a professor at McGill University and the CERN Department of Theoretical Physics in Switzerland, said in the statement. "The current data already hint at this behavior, and new data may be able to confirm it in the next few years."
If future measurements by pulsar timing arrays confirm the softening of the gravitational wave spectrum, scientists may finally be able to learn more about the elusive properties of dark matter from the behavior of some of the biggest giants in the universe.
Jonathan is an educator based in Hungary with a passion for astronomy. He enjoys communicating science stories in astrophysics and cosmology. He has a bachelor's degree in astrophysics from Cardiff University and a PhD in astronomy from Queen Mary University of London. In his spare time he enjoys hiking with his family and exploring the night sky.