World's thinnest electronic device is 2 atoms thick
The device uses quantum tunneling to encode data.
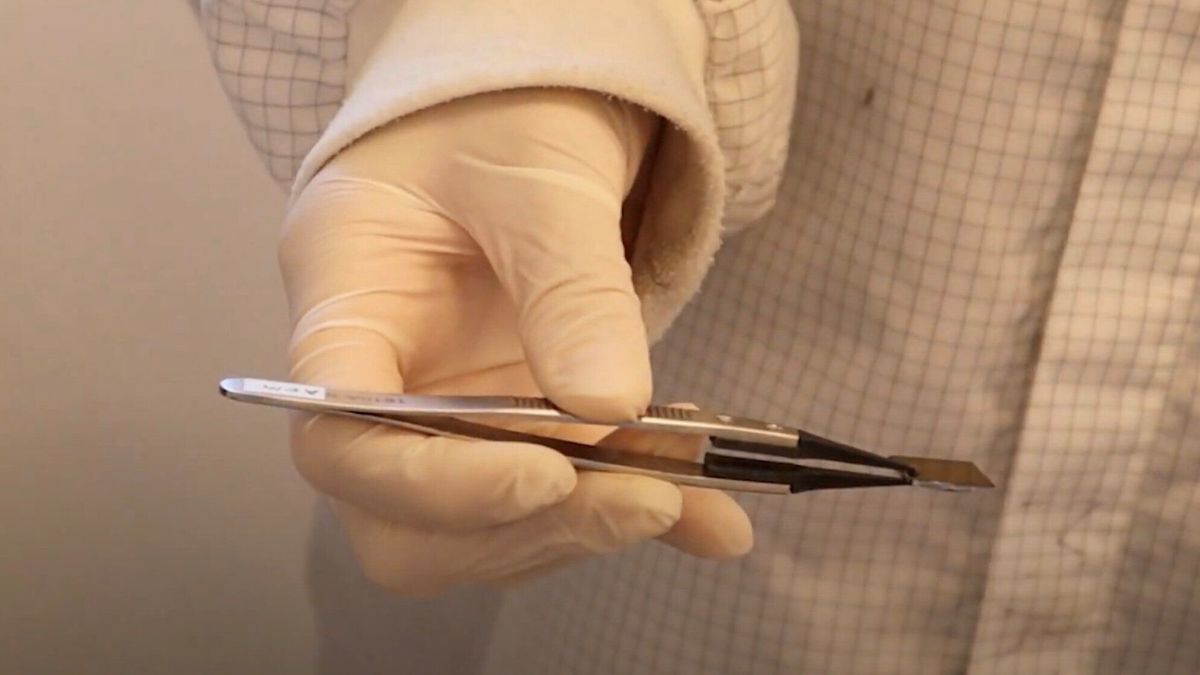
Scientists have developed the world’s thinnest piece of technology, a tiny device only two atoms thick that can be used to store electronic information.
The device consists of two layers, one made up of boron and the other of nitrogen, arranged in a repeating hexagonal structure. By taking advantage of a strange quantum mechanical effect called quantum tunneling, electrons from the boron and nitrogen atoms are able to zip across the gap between the two layers, changing the state of the device and allowing it to encode digital information.
This is similar to the way current state-of-the-art computing devices work. The hearts of computers contain many tiny crystals, each consisting of roughly a million atoms stacked in multiple, 100-atom layers. By shuttling electrons across gaps between the layers, computers are able to switch between the two binary states (0 and 1) that form the basis of the basic unit of digital information, the bit.
Related: 18 times quantum particles blew our minds
"In its natural three-dimensional state, this material (the crystal) is made up of a large number of layers placed on top of each other, with each layer rotated 180 degrees relative to its neighbors," Moshe Ben Shalom, a physicist at Tel Aviv University and a co-author of the study that developed the new technology, said in a statement. "In the lab, we were able to artificially stack the layers in a parallel configuration with no rotation, which hypothetically places atoms of the same kind in perfect overlap despite the strong repulsive force between them (resulting from their identical charges).”
Quantum tunneling enables particles — in this case electrons — to pass through seemingly impassable barriers. This is because in quantum physics, particles exist as both waves and particles simultaneously; those waves are the projected probabilities of the particle existing in a given space. Much like a wave smashing against a groin at sea will result in a smaller wave propagating to the other side, particles that exist as waves also have some probability of existing at the other side of a barrier.
It is this ability that allows electrons to leap between the device’s boron and nitrogen layers.
Sign up for the Live Science daily newsletter now
Get the world’s most fascinating discoveries delivered straight to your inbox.
In reality, the team said that the two layers do not perfectly align, instead preferring to slide slightly off center from one another so that the opposite charges of each layer overlap. This causes the free electrons (negatively charged) to move toward one layer and the positively charged atomic nuclei to the other, creating a small amount of electronic polarization — one side being positively charged and the other negatively charged — inside the device. By adjusting how one layer relates to the other, the polarization can be reversed — changing the device from one binary state to the other, and with it the stored information.
By reducing the size of the technology down to just two layers of atoms, the researchers could speed up the electron movement. Quicker electron movement could make future devices faster, less dense and more energy efficient.
Throughout the rise of computing in the late 20th and early 21st centuries, the growth of computer processing power was described by Moore's law, which says that the number of transistors that can fit on a chip doubles every two years, with an accompanying increase in performance. But as chip makers hit fundamental physical limits on how small transistors can get, this trend is slowing. The researchers hope that electronic chips based upon the new device’s design could change this slowdown.
"We hope that miniaturization and flipping (the polarization of the device) through sliding will improve today’s electronic devices, and moreover, allow other original ways of controlling information in future devices," lead author Maayan Vizner Stern, a doctoral candidate at Tel Aviv University, said in the statement.
The researchers published their findings June 25 in the journal Science.
Originally published on Live Science
Ben Turner is a U.K. based staff writer at Live Science. He covers physics and astronomy, among other topics like tech and climate change. He graduated from University College London with a degree in particle physics before training as a journalist. When he's not writing, Ben enjoys reading literature, playing the guitar and embarrassing himself with chess.